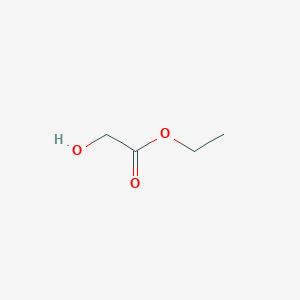
Glycolate d'éthyle
Vue d'ensemble
Description
Ethyl glycolate, also known as 2-hydroxyacetic acid ethyl ester, is an organic compound with the molecular formula C4H8O3. It is a colorless liquid with a mild, pleasant odor and is highly soluble in water and various organic solvents. Ethyl glycolate is primarily used as an intermediate in organic synthesis, particularly in the production of pharmaceuticals, agrochemicals, and other fine chemicals .
Applications De Recherche Scientifique
Ethyl glycolate is widely used in scientific research due to its versatility and reactivity. Some of its applications include:
Mécanisme D'action
Target of Action
Ethyl glycolate is primarily used as a building block for the preparation of medicinal compounds and agrochemicals . It serves as a starting reagent in the synthesis of various compounds .
Mode of Action
For instance, it is used as a substrate during the polycondensation of α-hydroxy acids by enzymes and polyethylene glycol (PEG)-modified enzymes .
Biochemical Pathways
Ethyl glycolate enters serine/glycine metabolism . The conversion of glycolate into glycine and serine helps ameliorate the drastically decreased NADPH/NADP+ and GSH/GSSG ratios induced by H2O2 treatment . This highlights the central role of serine/glycine metabolism as a major provider of reducing equivalents to maintain cellular antioxidant systems .
Result of Action
The molecular and cellular effects of ethyl glycolate’s action are largely dependent on its application. In the context of its use as a building block for medicinal compounds and agrochemicals, ethyl glycolate contributes to the synthesis of these compounds, thereby influencing their respective actions .
Action Environment
Environmental factors can influence the action, efficacy, and stability of ethyl glycolate. For instance, oxygen concentration has been identified as an important metabolic valve in the assimilation and use of ethylene glycol, a compound related to ethyl glycolate . .
Analyse Biochimique
Biochemical Properties
Ethyl glycolate participates in several biochemical reactions. It serves as a substrate during the polycondensation of α-hydroxy acids by enzymes and polyethylene glycol (PEG)-modified enzymes . The interactions of ethyl glycolate with these enzymes and biomolecules are crucial for its role in these reactions.
Cellular Effects
The cellular effects of ethyl glycolate are primarily related to its metabolic products. For instance, ethylene glycol, a related compound, is metabolized into glycolate, which can cause metabolic acidemia, neurotoxicity, and acute kidney injury
Molecular Mechanism
The molecular mechanism of ethyl glycolate’s action involves its metabolism by enzymes. It is believed that the rate-limiting step in this process is the activity of alcohol dehydrogenase . This enzyme catalyzes the oxidation of ethyl glycolate, leading to the production of glycolate, a metabolite with direct cellular toxicity .
Dosage Effects in Animal Models
Research on ethylene glycol poisoning suggests that the severity of symptoms is dose-dependent
Metabolic Pathways
Ethyl glycolate is involved in several metabolic pathways. For instance, it is used as a starting reagent in the synthesis of 2-ferrocenylfuran . It’s also a part of the glycolate pathway, where it is metabolized into glyoxylate .
Méthodes De Préparation
Synthetic Routes and Reaction Conditions
Ethyl glycolate can be synthesized through several methods. One common method involves the esterification of glycolic acid with ethanol in the presence of an acid catalyst such as sulfuric acid. The reaction is typically carried out under reflux conditions to drive the reaction to completion and achieve high yields .
Another method involves the hydrogenation of diethyl oxalate using a silver catalyst supported on silica (Ag/SiO2). This method has been shown to achieve high selectivity and conversion rates, making it suitable for industrial-scale production .
Industrial Production Methods
In industrial settings, ethyl glycolate is often produced by the esterification of glycolic acid with ethanol. The process involves the use of a strong acid catalyst and is carried out under controlled temperature and pressure conditions to optimize yield and purity. The resulting product is then purified through distillation to remove any unreacted starting materials and by-products .
Analyse Des Réactions Chimiques
Types of Reactions
Ethyl glycolate undergoes various chemical reactions, including:
Esterification: Reaction with alcohols to form esters.
Hydrolysis: Reaction with water to form glycolic acid and ethanol.
Oxidation: Reaction with oxidizing agents to form glycolic acid.
Reduction: Reaction with reducing agents to form ethylene glycol
Common Reagents and Conditions
Esterification: Typically involves an acid catalyst such as sulfuric acid and is carried out under reflux conditions.
Hydrolysis: Requires the presence of water and can be catalyzed by acids or bases.
Oxidation: Common oxidizing agents include hydrogen peroxide and potassium permanganate.
Reduction: Common reducing agents include lithium aluminum hydride and sodium borohydride.
Major Products Formed
Esterification: Forms various esters depending on the alcohol used.
Hydrolysis: Produces glycolic acid and ethanol.
Oxidation: Produces glycolic acid.
Reduction: Produces ethylene glycol
Comparaison Avec Des Composés Similaires
Ethyl glycolate is similar to other glycolate esters, such as methyl glycolate and benzyl glycolate. it is unique in its balance of reactivity and stability, making it particularly useful in a wide range of applications. Some similar compounds include:
Methyl glycolate: Used in similar applications but has different physical properties and reactivity.
Benzyl glycolate: Used as a fragrance ingredient and in organic synthesis
Ethyl glycolate’s unique combination of properties makes it a valuable compound in both research and industrial applications.
Propriétés
IUPAC Name |
ethyl 2-hydroxyacetate | |
---|---|---|
Source | PubChem | |
URL | https://pubchem.ncbi.nlm.nih.gov | |
Description | Data deposited in or computed by PubChem | |
InChI |
InChI=1S/C4H8O3/c1-2-7-4(6)3-5/h5H,2-3H2,1H3 | |
Source | PubChem | |
URL | https://pubchem.ncbi.nlm.nih.gov | |
Description | Data deposited in or computed by PubChem | |
InChI Key |
ZANNOFHADGWOLI-UHFFFAOYSA-N | |
Source | PubChem | |
URL | https://pubchem.ncbi.nlm.nih.gov | |
Description | Data deposited in or computed by PubChem | |
Canonical SMILES |
CCOC(=O)CO | |
Source | PubChem | |
URL | https://pubchem.ncbi.nlm.nih.gov | |
Description | Data deposited in or computed by PubChem | |
Molecular Formula |
C4H8O3 | |
Source | PubChem | |
URL | https://pubchem.ncbi.nlm.nih.gov | |
Description | Data deposited in or computed by PubChem | |
DSSTOX Substance ID |
DTXSID5060772 | |
Record name | Acetic acid, hydroxy-, ethyl ester | |
Source | EPA DSSTox | |
URL | https://comptox.epa.gov/dashboard/DTXSID5060772 | |
Description | DSSTox provides a high quality public chemistry resource for supporting improved predictive toxicology. | |
Molecular Weight |
104.10 g/mol | |
Source | PubChem | |
URL | https://pubchem.ncbi.nlm.nih.gov | |
Description | Data deposited in or computed by PubChem | |
Physical Description |
Clear colorless liquid; [Acros Organics MSDS] | |
Record name | Ethyl glycolate | |
Source | Haz-Map, Information on Hazardous Chemicals and Occupational Diseases | |
URL | https://haz-map.com/Agents/20781 | |
Description | Haz-Map® is an occupational health database designed for health and safety professionals and for consumers seeking information about the adverse effects of workplace exposures to chemical and biological agents. | |
Explanation | Copyright (c) 2022 Haz-Map(R). All rights reserved. Unless otherwise indicated, all materials from Haz-Map are copyrighted by Haz-Map(R). No part of these materials, either text or image may be used for any purpose other than for personal use. Therefore, reproduction, modification, storage in a retrieval system or retransmission, in any form or by any means, electronic, mechanical or otherwise, for reasons other than personal use, is strictly prohibited without prior written permission. | |
CAS No. |
623-50-7 | |
Record name | Ethyl glycolate | |
Source | CAS Common Chemistry | |
URL | https://commonchemistry.cas.org/detail?cas_rn=623-50-7 | |
Description | CAS Common Chemistry is an open community resource for accessing chemical information. Nearly 500,000 chemical substances from CAS REGISTRY cover areas of community interest, including common and frequently regulated chemicals, and those relevant to high school and undergraduate chemistry classes. This chemical information, curated by our expert scientists, is provided in alignment with our mission as a division of the American Chemical Society. | |
Explanation | The data from CAS Common Chemistry is provided under a CC-BY-NC 4.0 license, unless otherwise stated. | |
Record name | Ethyl glycolate | |
Source | ChemIDplus | |
URL | https://pubchem.ncbi.nlm.nih.gov/substance/?source=chemidplus&sourceid=0000623507 | |
Description | ChemIDplus is a free, web search system that provides access to the structure and nomenclature authority files used for the identification of chemical substances cited in National Library of Medicine (NLM) databases, including the TOXNET system. | |
Record name | Ethyl glycolate | |
Source | DTP/NCI | |
URL | https://dtp.cancer.gov/dtpstandard/servlet/dwindex?searchtype=NSC&outputformat=html&searchlist=8835 | |
Description | The NCI Development Therapeutics Program (DTP) provides services and resources to the academic and private-sector research communities worldwide to facilitate the discovery and development of new cancer therapeutic agents. | |
Explanation | Unless otherwise indicated, all text within NCI products is free of copyright and may be reused without our permission. Credit the National Cancer Institute as the source. | |
Record name | Acetic acid, 2-hydroxy-, ethyl ester | |
Source | EPA Chemicals under the TSCA | |
URL | https://www.epa.gov/chemicals-under-tsca | |
Description | EPA Chemicals under the Toxic Substances Control Act (TSCA) collection contains information on chemicals and their regulations under TSCA, including non-confidential content from the TSCA Chemical Substance Inventory and Chemical Data Reporting. | |
Record name | Acetic acid, hydroxy-, ethyl ester | |
Source | EPA DSSTox | |
URL | https://comptox.epa.gov/dashboard/DTXSID5060772 | |
Description | DSSTox provides a high quality public chemistry resource for supporting improved predictive toxicology. | |
Record name | Ethyl glycollate | |
Source | European Chemicals Agency (ECHA) | |
URL | https://echa.europa.eu/substance-information/-/substanceinfo/100.009.818 | |
Description | The European Chemicals Agency (ECHA) is an agency of the European Union which is the driving force among regulatory authorities in implementing the EU's groundbreaking chemicals legislation for the benefit of human health and the environment as well as for innovation and competitiveness. | |
Explanation | Use of the information, documents and data from the ECHA website is subject to the terms and conditions of this Legal Notice, and subject to other binding limitations provided for under applicable law, the information, documents and data made available on the ECHA website may be reproduced, distributed and/or used, totally or in part, for non-commercial purposes provided that ECHA is acknowledged as the source: "Source: European Chemicals Agency, http://echa.europa.eu/". Such acknowledgement must be included in each copy of the material. ECHA permits and encourages organisations and individuals to create links to the ECHA website under the following cumulative conditions: Links can only be made to webpages that provide a link to the Legal Notice page. | |
Record name | ETHYL GLYCOLATE | |
Source | FDA Global Substance Registration System (GSRS) | |
URL | https://gsrs.ncats.nih.gov/ginas/app/beta/substances/C306E91WFK | |
Description | The FDA Global Substance Registration System (GSRS) enables the efficient and accurate exchange of information on what substances are in regulated products. Instead of relying on names, which vary across regulatory domains, countries, and regions, the GSRS knowledge base makes it possible for substances to be defined by standardized, scientific descriptions. | |
Explanation | Unless otherwise noted, the contents of the FDA website (www.fda.gov), both text and graphics, are not copyrighted. They are in the public domain and may be republished, reprinted and otherwise used freely by anyone without the need to obtain permission from FDA. Credit to the U.S. Food and Drug Administration as the source is appreciated but not required. | |
Retrosynthesis Analysis
AI-Powered Synthesis Planning: Our tool employs the Template_relevance Pistachio, Template_relevance Bkms_metabolic, Template_relevance Pistachio_ringbreaker, Template_relevance Reaxys, Template_relevance Reaxys_biocatalysis model, leveraging a vast database of chemical reactions to predict feasible synthetic routes.
One-Step Synthesis Focus: Specifically designed for one-step synthesis, it provides concise and direct routes for your target compounds, streamlining the synthesis process.
Accurate Predictions: Utilizing the extensive PISTACHIO, BKMS_METABOLIC, PISTACHIO_RINGBREAKER, REAXYS, REAXYS_BIOCATALYSIS database, our tool offers high-accuracy predictions, reflecting the latest in chemical research and data.
Strategy Settings
Precursor scoring | Relevance Heuristic |
---|---|
Min. plausibility | 0.01 |
Model | Template_relevance |
Template Set | Pistachio/Bkms_metabolic/Pistachio_ringbreaker/Reaxys/Reaxys_biocatalysis |
Top-N result to add to graph | 6 |
Feasible Synthetic Routes
Q1: What is the molecular formula and weight of ethyl glycolate?
A1: Ethyl glycolate has the molecular formula C4H8O3 and a molecular weight of 104.10 g/mol.
Q2: How can ethyl glycolate be synthesized?
A2: Ethyl glycolate can be synthesized through various methods, including:
- Acid hydrolysis of ethyl diazoacetate: This method utilizes deuterated solvents and yields around 59% of deuterium-labeled ethyl glycolate. []
- Multi-step synthesis from glycine: This approach involves a three-step procedure starting with 13C2-glycine, resulting in a 35% yield of 13C2-labeled ethyl glycolate. []
- Reaction of 4-amino-3,5-dichloro-2,6-fluoro-2-pyridine with ethyl glycolate: This method, in the presence of sodium hydride, produces fluroxypyr ethyl ester with a 70% yield. []
Q3: Are there any spectroscopic data available for ethyl glycolate?
A3: Yes, ethyl glycolate has been characterized using various spectroscopic techniques. Researchers have investigated its microwave spectrum, revealing information about its conformational equilibrium. [] Additionally, 1H and 13C NMR, IR, UV-Vis, and HRMS analyses have been employed to fully characterize purified ethyl glycolate. []
Q4: Is ethyl glycolate stable under hydrolytic conditions?
A4: Ethyl glycolate exhibits hydrolytic degradation behavior. Studies have investigated its degradation in acidic, basic, and neutral media at 37°C when incorporated into polymers like poly[bis(methyl glycolate diethylamino)phosphazenes] (PMGDEAP) and poly[bis(ethyl glycolate diethylamino)phosphazenes] (PEGDEAP). []
Q5: What are the applications of ethyl glycolate in polymer chemistry?
A5: Ethyl glycolate serves as a valuable monomer in synthesizing various polymers:
- Biodegradable poly(ester hydrazide)s: Reacting ethyl glycolate with hydrazine produces 1,2-bisglycoylhydrazine, a monomer for creating these polymers through lipase-catalyzed synthesis. []
- Poly(PDL-co-GA) copolymers: Candida antarctica lipase B (CALB) effectively catalyzes the copolymerization of ω-pentadecalactone (PDL) and ethyl glycolate (EGA), forming these copolymers. []
- Poly(butylene-co-sebacate-co-glycolate) (PBSG) copolyesters: These copolyesters, synthesized via CALB-catalyzed polycondensation of ethyl glycolate, diethyl sebacate, and 1,4-butanediol, have potential as biodegradable carriers for drug delivery. []
Q6: Are there any specific applications for purified ethyl glycolate?
A6: Purified methyl phthalyl ethyl glycolate (MPEG) is used in various formulations. The development of a flash column chromatography purification method for MPEG allows for the establishment of analytically pure standards, ensuring better quality control in formulations containing MPEG. []
Q7: What is the role of ethyl glycolate in the synthesis of other compounds?
A7: Ethyl glycolate acts as a starting material in various syntheses:
- γ-ketocrotonates: In situ oxidation-Wittig methodology, applied to α-hydroxyketones with ethyl glycolate, provides a simple and efficient route to γ-ketocrotonates. [, ]
- Fluoroethanol: No-carrier-added fluorine-18 fluoroethanol can be synthesized using ethyl glycolate as a precursor through fluoride-ion displacement on α-p-toluene sulfonyl ethyl glycolate followed by reduction. [, ]
- 4′-Cyclopropylated carbovir analogues: Ethyl glycolate serves as a starting point for the synthesis of these analogues through Johnson's orthoester rearrangement and ring-closing metathesis. []
Q8: Can ethyl glycolate be produced through hydrogenation reactions?
A8: Yes, ethyl glycolate is a product of diethyl oxalate hydrogenation. This reaction utilizes various catalysts and has garnered significant research interest as a potential environmentally friendly method for producing ethyl glycolate. [] Research focuses on developing efficient catalysts for industrial applications. [] The thermodynamics of this reaction, particularly the enthalpy, entropy, and equilibrium constant, have been studied to optimize the process. []
Q9: Are there any specific catalysts used for diethyl oxalate hydrogenation to ethyl glycolate?
A9: Research highlights the use of various catalysts for this reaction:
- Supported Ni catalysts: These catalysts, particularly when modulated with phosphorus, show promising activity and selectivity for ethyl glycolate production. []
- Highly dispersed Ag/SiO2 catalyst: This catalyst, characterized by weak metal-support interaction, exhibits good performance in diethyl oxalate hydrogenation to ethyl glycolate. []
- Single-atom silver-manganese nanocatalysts: This type of catalyst, designed with atom-economy principles, displays temperature-controlled selectivity for either ethyl glycolate or acetaldehyde diethyl acetal production. []
Q10: Are there computational studies on ethyl glycolate?
A10: Yes, computational chemistry tools have been employed to study ethyl glycolate. Ab initio computations, combined with microwave spectroscopy data, help analyze the conformational equilibrium of ethyl glycolate in the gas phase. []
Q11: What is the environmental fate of ethyl glycolate?
A11: While specific information about ethyl glycolate's environmental degradation is limited within the provided research papers, its use in synthesizing biodegradable polymers like poly(ester hydrazide)s [] and poly(butylene-co-sebacate-co-glycolate) copolyesters [] suggests potential for biodegradation. Further research is needed to understand its complete environmental impact and degradation pathways.
Q12: Has ethyl glycolate been used in the study of insects?
A12: Yes, ethyl glycolate has been identified as a compound found in the Mediterranean fruit fly (Ceratitis capitata). Researchers utilize techniques like Direct Immersion-Solid Phase Microextraction (DI-SPME) and Gas Chromatography-Mass Spectrometry (GC/MS) to extract and analyze ethyl glycolate and other compounds from these flies. This helps in understanding biological changes in the flies during mating and development. [, ]
Avertissement et informations sur les produits de recherche in vitro
Veuillez noter que tous les articles et informations sur les produits présentés sur BenchChem sont destinés uniquement à des fins informatives. Les produits disponibles à l'achat sur BenchChem sont spécifiquement conçus pour des études in vitro, qui sont réalisées en dehors des organismes vivants. Les études in vitro, dérivées du terme latin "in verre", impliquent des expériences réalisées dans des environnements de laboratoire contrôlés à l'aide de cellules ou de tissus. Il est important de noter que ces produits ne sont pas classés comme médicaments et n'ont pas reçu l'approbation de la FDA pour la prévention, le traitement ou la guérison de toute condition médicale, affection ou maladie. Nous devons souligner que toute forme d'introduction corporelle de ces produits chez les humains ou les animaux est strictement interdite par la loi. Il est essentiel de respecter ces directives pour assurer la conformité aux normes légales et éthiques en matière de recherche et d'expérimentation.