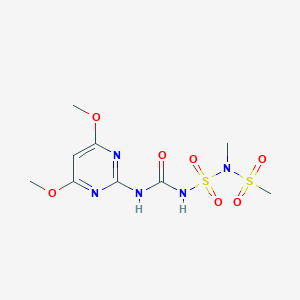
Amidosulfuron
Vue d'ensemble
Description
L’amidosulfuron est un herbicide à large spectre principalement utilisé pour contrôler les mauvaises herbes à feuilles larges. Il appartient à la famille des herbicides sulfonylurées, connus pour leur forte solubilité dans l’eau et leur potentiel de lessivage dans les eaux souterraines. L’this compound est volatil et présente une toxicité modérée pour la plupart des espèces terrestres et aquatiques. Il n’est pas persistant dans les systèmes du sol, mais peut persister dans l’eau dans certaines conditions .
Applications De Recherche Scientifique
Amidosulfuron has a wide range of scientific research applications, including:
Chemistry: Used as a model compound to study the behavior of sulfonylurea herbicides in various chemical reactions.
Biology: Investigated for its effects on plant physiology and metabolism, particularly in relation to its herbicidal activity.
Medicine: Explored for potential therapeutic applications due to its ability to inhibit specific enzymes.
Industry: Utilized in agricultural practices to control broad-leaved weeds in crops such as cereals, linseed, flax, and grassland
Mécanisme D'action
L’amidosulfuron exerce ses effets herbicides en inhibant l’enzyme acétolactate synthase, qui est cruciale pour la synthèse des acides aminés à chaîne ramifiée dans les plantes. Cette inhibition perturbe la synthèse des protéines, ce qui entraîne la mort des mauvaises herbes ciblées. Les cibles et les voies moléculaires impliquées comprennent la liaison de l’this compound au site actif de l’acétolactate synthase, empêchant l’enzyme de catalyser ses réactions normales .
Composés Similaires :
- Méthyle de tribenuron
- Méthyle de metsulfuron
- Cinosulfuron
- Pyrazosulfuron-éthyle
- Imazosulfuron
Comparaison : L’this compound est unique parmi les herbicides sulfonylurées en raison de ses caractéristiques structurelles spécifiques et de son mode d’action. Contrairement aux autres sulfonylurées, l’this compound ne contient pas de sulfonamide aromatique substitué. Malgré cette déviation structurelle, il inhibe toujours l’acétolactate synthase, de manière similaire aux autres herbicides sulfonylurées. Cette structure unique contribue à ses propriétés herbicides spécifiques et à son efficacité dans le contrôle d’un large éventail de mauvaises herbes à feuilles larges .
Analyse Biochimique
Biochemical Properties
Amidosulfuron interacts with various enzymes and proteins in plants. It is primarily absorbed by the leaves of plants but is also absorbed through the roots . It has special activity against Galium aparine at rates as low as 15 g a.i./ha .
Cellular Effects
This compound has significant effects on various types of cells and cellular processes. It causes chloroplasts in leaf and anther epidermis, and tapetal plastids to deform . Both tapetal cell and uni-nucleate microspore show autophagic vacuoles and degenerate quickly after exposure to this compound .
Molecular Mechanism
This compound exerts its effects at the molecular level primarily by inhibiting the enzyme Acetolactate synthase (ALS), which is a key enzyme in the biosynthesis of branched-chain amino acids . This inhibition leads to a reduction in photosynthetic rate and the contents of leaf chlorophyll, soluble sugar, and pyruvate .
Temporal Effects in Laboratory Settings
The degradation of this compound in soil is strongly dependent on temperature and moisture levels. In controlled laboratory experiments, first-order half-lives ranged from 26 ± 3 days to 79 ± 2 days in three Canadian soils at 20°C .
Metabolic Pathways
This compound is involved in the metabolic pathway related to the biosynthesis of branched-chain amino acids . It inhibits the enzyme Acetolactate synthase (ALS), disrupting this pathway .
Transport and Distribution
This compound is primarily absorbed by the leaves of plants but is also absorbed through the roots . The specific transporters or binding proteins it interacts with are not currently known.
Subcellular Localization
It is known to cause deformation of chloroplasts in leaf and anther epidermis, and tapetal plastids . This suggests that this compound may localize to these organelles within the cell.
Méthodes De Préparation
Voies de Synthèse et Conditions de Réaction : L’amidosulfuron est synthétisé par une série de réactions chimiques impliquant des intermédiaires sulfonyluréesLes conditions de réaction nécessitent souvent des températures contrôlées et l’utilisation de solvants pour faciliter les réactions .
Méthodes de Production Industrielle : La production industrielle de l’this compound implique une synthèse chimique à grande échelle utilisant des voies de réaction similaires à celles de la synthèse en laboratoire. Le processus est optimisé pour un rendement et une pureté élevés, avec des mesures de contrôle qualité strictes pour garantir la cohérence et la sécurité. Les installations de production sont équipées de technologies de pointe pour gérer la nature volatile et potentiellement dangereuse des produits chimiques impliqués .
Analyse Des Réactions Chimiques
Types de Réactions : L’amidosulfuron subit diverses réactions chimiques, notamment :
Oxydation : Implique l’ajout d’oxygène ou le retrait d’hydrogène.
Réduction : Implique l’ajout d’hydrogène ou le retrait d’oxygène.
Substitution : Implique le remplacement d’un groupe fonctionnel par un autre.
Réactifs et Conditions Courants :
Oxydation : Les oxydants courants comprennent le permanganate de potassium et le peroxyde d’hydrogène.
Réduction : Les réducteurs courants comprennent le borohydrure de sodium et l’hydrure de lithium et d’aluminium.
Substitution : Les réactifs courants comprennent les halogènes et les nucléophiles dans des conditions de température et de pH contrôlées.
Principaux Produits Formés : Les principaux produits formés à partir de ces réactions dépendent des réactifs et des conditions spécifiques utilisés. Par exemple, l’oxydation peut produire des acides sulfoniques, tandis que la réduction peut produire des amines .
4. Applications de la Recherche Scientifique
L’this compound a un large éventail d’applications de recherche scientifique, notamment :
Chimie : Utilisé comme composé modèle pour étudier le comportement des herbicides sulfonylurées dans diverses réactions chimiques.
Biologie : Investigé pour ses effets sur la physiologie et le métabolisme des plantes, en particulier en relation avec son activité herbicide.
Médecine : Exploré pour ses applications thérapeutiques potentielles en raison de sa capacité à inhiber des enzymes spécifiques.
Industrie : Utilisé dans les pratiques agricoles pour contrôler les mauvaises herbes à feuilles larges dans les cultures telles que les céréales, le lin, le lin et les prairies
Comparaison Avec Des Composés Similaires
- Tribenuron Methyl
- Metsulfuron Methyl
- Cinosulfuron
- Pyrazosulfuron Ethyl
- Imazosulfuron
Comparison: Amidosulfuron is unique among sulfonylurea herbicides due to its specific structural features and mode of action. Unlike other sulfonylureas, this compound does not contain a substituted aromatic sulfonamide. Despite this structural deviation, it still inhibits acetolactate synthase, similar to other sulfonylurea herbicides. This unique structure contributes to its specific herbicidal properties and effectiveness in controlling a wide range of broad-leaved weeds .
Propriétés
IUPAC Name |
1-(4,6-dimethoxypyrimidin-2-yl)-3-[methyl(methylsulfonyl)sulfamoyl]urea | |
---|---|---|
Source | PubChem | |
URL | https://pubchem.ncbi.nlm.nih.gov | |
Description | Data deposited in or computed by PubChem | |
InChI |
InChI=1S/C9H15N5O7S2/c1-14(22(4,16)17)23(18,19)13-9(15)12-8-10-6(20-2)5-7(11-8)21-3/h5H,1-4H3,(H2,10,11,12,13,15) | |
Source | PubChem | |
URL | https://pubchem.ncbi.nlm.nih.gov | |
Description | Data deposited in or computed by PubChem | |
InChI Key |
CTTHWASMBLQOFR-UHFFFAOYSA-N | |
Source | PubChem | |
URL | https://pubchem.ncbi.nlm.nih.gov | |
Description | Data deposited in or computed by PubChem | |
Canonical SMILES |
CN(S(=O)(=O)C)S(=O)(=O)NC(=O)NC1=NC(=CC(=N1)OC)OC | |
Source | PubChem | |
URL | https://pubchem.ncbi.nlm.nih.gov | |
Description | Data deposited in or computed by PubChem | |
Molecular Formula |
C9H15N5O7S2 | |
Source | PubChem | |
URL | https://pubchem.ncbi.nlm.nih.gov | |
Description | Data deposited in or computed by PubChem | |
DSSTOX Substance ID |
DTXSID7057945 | |
Record name | Amidosulfuron | |
Source | EPA DSSTox | |
URL | https://comptox.epa.gov/dashboard/DTXSID7057945 | |
Description | DSSTox provides a high quality public chemistry resource for supporting improved predictive toxicology. | |
Molecular Weight |
369.4 g/mol | |
Source | PubChem | |
URL | https://pubchem.ncbi.nlm.nih.gov | |
Description | Data deposited in or computed by PubChem | |
CAS No. |
120923-37-7 | |
Record name | Amidosulfuron | |
Source | CAS Common Chemistry | |
URL | https://commonchemistry.cas.org/detail?cas_rn=120923-37-7 | |
Description | CAS Common Chemistry is an open community resource for accessing chemical information. Nearly 500,000 chemical substances from CAS REGISTRY cover areas of community interest, including common and frequently regulated chemicals, and those relevant to high school and undergraduate chemistry classes. This chemical information, curated by our expert scientists, is provided in alignment with our mission as a division of the American Chemical Society. | |
Explanation | The data from CAS Common Chemistry is provided under a CC-BY-NC 4.0 license, unless otherwise stated. | |
Record name | Amidosulfuron [ISO] | |
Source | ChemIDplus | |
URL | https://pubchem.ncbi.nlm.nih.gov/substance/?source=chemidplus&sourceid=0120923377 | |
Description | ChemIDplus is a free, web search system that provides access to the structure and nomenclature authority files used for the identification of chemical substances cited in National Library of Medicine (NLM) databases, including the TOXNET system. | |
Record name | Amidosulfuron | |
Source | EPA DSSTox | |
URL | https://comptox.epa.gov/dashboard/DTXSID7057945 | |
Description | DSSTox provides a high quality public chemistry resource for supporting improved predictive toxicology. | |
Record name | 3,5-Dithia-2,4-diazahexanamide, N-(4,6-dimethoxy-2-pyrimidinyl)-4-methyl-, 3,3,5,5-tetraoxide | |
Source | European Chemicals Agency (ECHA) | |
URL | https://echa.europa.eu/substance-information/-/substanceinfo/100.129.777 | |
Description | The European Chemicals Agency (ECHA) is an agency of the European Union which is the driving force among regulatory authorities in implementing the EU's groundbreaking chemicals legislation for the benefit of human health and the environment as well as for innovation and competitiveness. | |
Explanation | Use of the information, documents and data from the ECHA website is subject to the terms and conditions of this Legal Notice, and subject to other binding limitations provided for under applicable law, the information, documents and data made available on the ECHA website may be reproduced, distributed and/or used, totally or in part, for non-commercial purposes provided that ECHA is acknowledged as the source: "Source: European Chemicals Agency, http://echa.europa.eu/". Such acknowledgement must be included in each copy of the material. ECHA permits and encourages organisations and individuals to create links to the ECHA website under the following cumulative conditions: Links can only be made to webpages that provide a link to the Legal Notice page. | |
Record name | AMIDOSULFURON | |
Source | FDA Global Substance Registration System (GSRS) | |
URL | https://gsrs.ncats.nih.gov/ginas/app/beta/substances/6ZNU868K0S | |
Description | The FDA Global Substance Registration System (GSRS) enables the efficient and accurate exchange of information on what substances are in regulated products. Instead of relying on names, which vary across regulatory domains, countries, and regions, the GSRS knowledge base makes it possible for substances to be defined by standardized, scientific descriptions. | |
Explanation | Unless otherwise noted, the contents of the FDA website (www.fda.gov), both text and graphics, are not copyrighted. They are in the public domain and may be republished, reprinted and otherwise used freely by anyone without the need to obtain permission from FDA. Credit to the U.S. Food and Drug Administration as the source is appreciated but not required. | |
Synthesis routes and methods I
Procedure details
Synthesis routes and methods II
Procedure details
Synthesis routes and methods III
Procedure details
Synthesis routes and methods IV
Procedure details
Synthesis routes and methods V
Procedure details
Retrosynthesis Analysis
AI-Powered Synthesis Planning: Our tool employs the Template_relevance Pistachio, Template_relevance Bkms_metabolic, Template_relevance Pistachio_ringbreaker, Template_relevance Reaxys, Template_relevance Reaxys_biocatalysis model, leveraging a vast database of chemical reactions to predict feasible synthetic routes.
One-Step Synthesis Focus: Specifically designed for one-step synthesis, it provides concise and direct routes for your target compounds, streamlining the synthesis process.
Accurate Predictions: Utilizing the extensive PISTACHIO, BKMS_METABOLIC, PISTACHIO_RINGBREAKER, REAXYS, REAXYS_BIOCATALYSIS database, our tool offers high-accuracy predictions, reflecting the latest in chemical research and data.
Strategy Settings
Precursor scoring | Relevance Heuristic |
---|---|
Min. plausibility | 0.01 |
Model | Template_relevance |
Template Set | Pistachio/Bkms_metabolic/Pistachio_ringbreaker/Reaxys/Reaxys_biocatalysis |
Top-N result to add to graph | 6 |
Feasible Synthetic Routes
Q1: What is the primary target of amidosulfuron in plants?
A1: this compound, similar to other sulfonylurea herbicides, primarily targets acetolactate synthase (ALS) in plants. [] ALS is a key enzyme in the biosynthesis pathway of branched-chain amino acids, namely valine, leucine, and isoleucine. []
Q2: How does this compound interact with its target enzyme?
A2: this compound binds to ALS and inhibits its activity. This binding is time-dependent and accumulative, leading to the formation of a peracetate adduct with the enzyme's cofactor, thiamine diphosphate (ThDP). []
Q3: What are the downstream effects of this compound's inhibition of ALS?
A3: Inhibition of ALS by this compound disrupts the biosynthesis of branched-chain amino acids. This disruption leads to a cascade of effects, including reduced photosynthesis rates, chlorophyll content, and soluble sugar levels in plants. [] Additionally, alterations in the content of free amino acids and the appearance of autophagic vacuoles in cells are observed. [] Ultimately, these effects contribute to the death of susceptible plants.
Q4: What is the molecular formula and weight of this compound?
A4: Unfortunately, the provided research excerpts do not explicitly state the molecular formula and weight of this compound. To obtain this information, please refer to publicly available chemical databases or the this compound product safety data sheet.
Q5: Does the formulation of this compound influence its efficacy in weed control?
A5: Yes, studies show that the formulation of this compound can influence its efficacy. For instance, an oil dispersion (OD) formulation of an this compound and iodosulfuron-methyl-sodium mixture demonstrated greater sensitivity in controlling certain weed species compared to a water-dispersible granule (WG) formulation, particularly under specific environmental conditions. [, ]
Q6: How do environmental conditions affect the efficacy of this compound?
A6: Environmental factors such as temperature, relative humidity, and soil moisture can influence the efficacy of this compound. Research indicates that the efficacy of a WG formulation is more susceptible to these factors compared to an OD formulation. [] For example, high relative humidity and soil moisture generally led to better control of certain weed species with the WG formulation. []
Q7: Does this compound exhibit any catalytic properties?
A7: this compound is primarily known for its herbicidal activity, acting as an enzyme inhibitor rather than a catalyst. The research provided does not mention any catalytic properties associated with this compound.
Q8: Have computational methods been employed to study this compound's interactions with ALS?
A8: Yes, molecular docking studies have been conducted to investigate the interactions between this compound and ALS. These studies confirm that this compound effectively inhibits the binding of the enzyme's cofactor, TDL (lactyl-ThDP), to ALS. []
Q9: How does the structure of this compound compare to other sulfonylurea herbicides, and what is the impact on its activity?
A9: this compound, unlike many sulfonylurea herbicides, lacks a second aromatic ring in its structure. [] This structural difference results in fewer interactions with the ALS enzyme, leading to a relatively lower binding affinity compared to herbicides with two heteroaromatic rings. []
Q10: How does the stability of this compound vary with pH and temperature?
A10: this compound undergoes hydrolysis, and its degradation rate is influenced by both pH and temperature. [] The research indicates that hydrolysis follows first-order kinetics and is faster in acidic conditions (lower pH) and at higher temperatures. []
Q11: What analytical techniques are commonly employed for the detection and quantification of this compound?
A11: Several analytical techniques are used for the analysis of this compound, including:
Q12: What are the primary degradation pathways of this compound in the environment?
A12: Research indicates that this compound primarily degrades through hydrolysis in the environment. [, ] The rate of hydrolysis is affected by factors like soil pH, temperature, and moisture. [, ] Studies also demonstrate that microbial activity in the soil contributes to the degradation of this compound. [, ]
Q13: What are the identified degradation products of this compound?
A13: Studies employing simulated sunlight irradiation identified several degradation products of this compound in aqueous solutions. [] These products mainly result from the loss of molecules such as methylsulfamic acid, sulfocarbamic acid, carbamic acid, methyl(methylsulfonyl)sulfamic acid, N-methylmethanesulfonamide, and sulfonic acid. [] Additionally, processes like O- and S-demethylation and hydroxylation contribute to the formation of degradation products. []
Q14: How does this compound impact non-target organisms?
A14: Research shows that this compound can negatively impact non-target organisms. For example, exposure to this compound caused significant growth inhibition, reduced biomass, decreased photosynthetic pigment content, and increased oxidative stress markers in the aquatic macrophyte Lemna minor. [, ]
Q15: How does this compound behave in different soil types?
A15: The degradation and persistence of this compound vary depending on the soil type. Studies in Saskatchewan soils found that this compound had a longer half-life in clay soil compared to loamy sand soil under controlled laboratory conditions. [] This difference in degradation is attributed to factors like soil pH, organic matter content, and microbial activity. []
Avertissement et informations sur les produits de recherche in vitro
Veuillez noter que tous les articles et informations sur les produits présentés sur BenchChem sont destinés uniquement à des fins informatives. Les produits disponibles à l'achat sur BenchChem sont spécifiquement conçus pour des études in vitro, qui sont réalisées en dehors des organismes vivants. Les études in vitro, dérivées du terme latin "in verre", impliquent des expériences réalisées dans des environnements de laboratoire contrôlés à l'aide de cellules ou de tissus. Il est important de noter que ces produits ne sont pas classés comme médicaments et n'ont pas reçu l'approbation de la FDA pour la prévention, le traitement ou la guérison de toute condition médicale, affection ou maladie. Nous devons souligner que toute forme d'introduction corporelle de ces produits chez les humains ou les animaux est strictement interdite par la loi. Il est essentiel de respecter ces directives pour assurer la conformité aux normes légales et éthiques en matière de recherche et d'expérimentation.