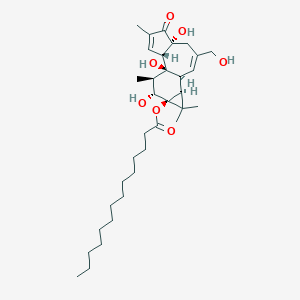
Phorbol 13-myristate
Vue d'ensemble
Description
Phorbol 13-myristate (commonly referred to as phorbol 12-myristate 13-acetate, PMA) is a phorbol ester derived from croton oil. It is a potent activator of protein kinase C (PKC), a key enzyme in cellular signaling pathways regulating proliferation, differentiation, and apoptosis . PMA is structurally characterized by a diterpene core with esterified acyl groups at positions 12 (myristate) and 13 (acetate) (Figure 1). Its biological activity is highly dependent on these substitutions, as modifications alter binding affinity to PKC isoforms and downstream effects . PMA is widely used in research to model PKC-driven processes, including immune cell activation, tumor promotion, and gene regulation .
Méthodes De Préparation
Synthetic Preparation Strategies
Natural Extraction vs. Synthetic Synthesis
PMA is structurally derived from phorbol, a diterpene found in the seeds of Croton tiglium (croton oil) . However, natural extraction is impractical for large-scale production due to low yields and the complexity of isolating PMA from other phorbol esters. Consequently, synthetic routes are preferred, though they present significant challenges.
Phorbol’s core structure contains four hydroxyl groups at positions 4, 9, 12, and 13, necessitating selective esterification at the 12- and 13-positions to produce PMA . This requires protective group strategies to prevent undesired reactions. A typical synthetic pathway involves:
-
Protection of Hydroxyl Groups : Temporary blocking of hydroxyls at positions 4 and 9 using tert-butyldimethylsilyl (TBDMS) or acetyl groups.
-
Esterification :
-
Myristoylation : Reaction of the 12-hydroxyl group with myristoyl chloride in the presence of a base (e.g., pyridine).
-
Acetylation : Treatment of the 13-hydroxyl group with acetic anhydride.
-
-
Deprotection : Removal of protective groups under mild acidic or basic conditions to yield PMA .
Despite this theoretical framework, detailed synthetic protocols remain proprietary or unpublished in open literature, likely due to the compound’s commercial value and technical complexity .
Purification and Quality Control
Crude PMA synthesis yields a mixture of esters, necessitating advanced purification techniques. Key methods include:
Purified PMA is characterized by a melting point of 250–252°C (decomposition) and a molecular weight of 616.8 g/mol . Commercial suppliers typically certify purity via HPLC, with grades exceeding 95% .
Preparation of Stock Solutions
PMA’s hydrophobicity necessitates dissolution in organic solvents before aqueous dilution. Standard protocols include:
Solvent | Stock Concentration | Storage | Stability |
---|---|---|---|
DMSO | 10–100 mM | -20°C, desiccated, dark | 1 month (aliquoted) |
Ethanol | ≤40 mM | -20°C, desiccated | 2 weeks |
For cell culture applications, working solutions are prepared by diluting stocks into media, ensuring final DMSO concentrations ≤0.1% to avoid cytotoxicity . Fresh preparation is recommended, though aliquots stored at -20°C retain activity for short-term use .
Form | Storage Conditions | Shelf Life |
---|---|---|
Lyophilized solid | -20°C, desiccated, protected from light | 12 months |
DMSO solution | -20°C, aliquoted | 1 month |
Degradation products, including free phorbol and myristic acid, may form under suboptimal conditions, necessitating periodic HPLC reanalysis for critical applications .
Analytical Methods for Characterization
Structural Confirmation
-
Nuclear Magnetic Resonance (NMR) :
-
Mass Spectrometry (MS) :
Purity Assessment
-
High-Performance Liquid Chromatography (HPLC) :
Applications in Research Settings
While beyond preparation methods, PMA’s utility underscores the importance of rigorous synthesis and handling:
Analyse Des Réactions Chimiques
Types of Reactions
This compound can undergo various chemical reactions, including:
Oxidation: The hydroxyl groups can be oxidized to form ketones or aldehydes.
Reduction: The ketone group can be reduced to form secondary alcohols.
Substitution: The hydroxyl groups can be substituted with other functional groups through nucleophilic substitution reactions.
Common Reagents and Conditions
Oxidation: Common oxidizing agents include potassium permanganate (KMnO₄) and chromium trioxide (CrO₃).
Reduction: Reducing agents such as lithium aluminum hydride (LiAlH₄) and sodium borohydride (NaBH₄) are typically used.
Substitution: Nucleophiles such as halides (e.g., Cl⁻, Br⁻) and amines (e.g., NH₂⁻) can be used under basic conditions.
Major Products
The major products formed from these reactions depend on the specific reagents and conditions used. For example, oxidation of the hydroxyl groups may yield ketones or aldehydes, while reduction of the ketone group may produce secondary alcohols.
Applications De Recherche Scientifique
Molecular Biology Applications
Cell Growth and Differentiation
PMA is widely used to study the mechanisms of cell growth and differentiation. It activates PKC, which plays a crucial role in signaling pathways that regulate cellular functions. For instance, PMA has been shown to induce differentiation of THP-1 monocytes into macrophages, making it a valuable tool in immunological research .
Gene Expression Studies
PMA influences gene transcription through the activation of various transcription factors, including NF-κB. This property makes PMA an essential reagent for studying cytokine-mediated regulation of gene expression .
Cytokine Production
In research settings, PMA is utilized to stimulate interleukin-2 production in Jurkat cells and other immune cells. This application is critical for understanding T-cell activation and function .
Cancer Research
Tumor Promotion Studies
PMA is recognized as a potent tumor promoter in experimental models, particularly in mouse skin carcinogenesis studies. Its ability to activate PKC at nanomolar concentrations has made it a standard compound for investigating tumorigenesis mechanisms .
Metastatic Activity Investigation
Research indicates that PMA can enhance the metastatic potential of various cancer cell lines by modulating cell adhesion and migration properties through PKC activation . This application is vital for developing anti-metastatic therapies.
Immunology Applications
B Cell Activation and Differentiation
PMA has been shown to enhance IL-21-induced differentiation of pre-activated B cells into granzyme B-producing cells (GraB cells). This application suggests potential therapeutic uses in autoimmune diseases and hematological disorders .
Regulatory T Cell Studies
The compound has been employed in studies exploring the role of regulatory T cells in immune responses, providing insights into their function and potential manipulation for therapeutic purposes .
Toxicological Studies
Toxicity Assessment
PMA's inflammatory properties have led to its use in toxicity studies. Research has demonstrated that PMA induces chromosomal aberrations and other toxic effects in various model systems, highlighting its potential risks in biological contexts .
Comprehensive Data Table
Case Studies
- Idiopathic Pulmonary Fibrosis (IPF) : In a study investigating the role of osteopontin in IPF, researchers used PMA to stimulate cellular responses associated with fibrosis. The findings suggested that PMA-induced pathways could be targeted for therapeutic interventions in IPF .
- Metastatic Mechanisms : A study on melanoma cells demonstrated that PMA enhances metastatic behavior through specific PKC-mediated pathways. This research provides insights into how PMA can be utilized to explore metastatic processes in cancer biology .
- Regulatory B Cells : Recent findings indicate that PMA can be combined with other stimuli to enhance the differentiation of regulatory B cells, suggesting its potential use in therapies aimed at modulating immune responses in autoimmune diseases .
Mécanisme D'action
The mechanism of action of [(1S,2S,6R,10S,11S,13S,14R,15R)-1,6,14-Trihydroxy-8-(hydroxymethyl)-4,12,12,15-tetramethyl-5-oxo-13-tetracyclo[8.5.0.02,6.011,13]pentadeca-3,8-dienyl] tetradecanoate involves its interaction with specific molecular targets and pathways. The hydroxyl and hydroxymethyl groups may participate in hydrogen bonding and other interactions with proteins and enzymes, modulating their activity and leading to various biological effects.
Comparaison Avec Des Composés Similaires
Comparison with Similar Phorbol Esters
Structural Analogues and Binding Affinities
Phorbol esters vary in their acyl chain substitutions, which critically influence their biological potency and receptor interactions. Key analogues include:
- PMA vs. PDBU : PMA exhibits 100-fold higher binding affinity to PKC in mouse brain than PDBU, likely due to its longer myristate chain enhancing membrane interaction .
- Prostratin : Lacks the 12-hydroxyl group, reducing tumor-promoting activity but retaining PKC activation. It antagonizes PMA-induced hyperplasia and ornithine decarboxylase induction in mouse skin .
- Resiniferatoxin: Despite structural similarity, it primarily activates TRPV1 receptors and shows weak PKC binding, explaining its divergent inflammatory and non-promoting properties .
Functional Antagonism and Therapeutic Implications
- Prostratin and dPP: These non-promoting phorbol esters inhibit PMA-induced tumor promotion in mouse skin by blocking hyperplasia (96% reduction in papillomas at 2.56 μmol) .
- Resiniferatoxin: Exhibits 100-fold higher inflammation in mouse ears than PMA but fails to promote tumors, underscoring the dissociation between PKC activation and carcinogenesis .
Mechanistic Insights from Structural Modifications
- Acyl Chain Length : Longer acyl chains (e.g., myristate in PMA vs. butyrate in PDBU) enhance membrane localization and PKCδ translocation to the plasma membrane .
- 12-Hydroxyl Group : Deletion (as in prostratin) reduces tumor-promoting activity but preserves anti-viral effects, suggesting structural determinants for functional specificity .
- Stereochemistry: 4α-phorbol derivatives (e.g., 4α-phorbol 12,13-didecanoate) lack biological activity, emphasizing the necessity of the 4β orientation for PKC binding .
Activité Biologique
Phorbol 13-myristate, commonly referred to as phorbol 12-myristate 13-acetate (PMA), is a potent tumor promoter and a highly effective activator of protein kinase C (PKC). Its biological activity has been extensively studied across various models, revealing significant implications for cell signaling, inflammation, and cancer research.
Phorbol esters, including PMA, are structurally similar to diacylglycerol (DAG), a natural second messenger involved in cellular signaling. PMA activates PKC by binding to its C1 domains, promoting the translocation of PKC isoforms to the cell membrane, where they become active. This activation leads to various downstream effects, including changes in gene expression and cell proliferation.
Biological Effects
- Tumor Promotion : PMA is recognized as one of the most effective tumor promoters. It induces cellular proliferation and differentiation in various cell types, including fibroblasts and epithelial cells. The compound has been shown to enhance the expression of oncogenes and inhibit apoptosis, thereby contributing to tumorigenesis .
- Inflammation : PMA is also known for its pro-inflammatory properties. It induces the release of cytokines and chemokines, leading to an inflammatory response that can mimic conditions such as psoriasis in animal models . Studies have demonstrated that PMA treatment can cause significant inflammatory responses in human keratinocyte cells and zebrafish embryos, resulting in chromosomal aberrations and developmental deformities .
- Neurotoxicity : Research indicates that PMA can adversely affect brain development in neonatal rodents. Exposure to PMA results in neurodegeneration and altered inflammatory responses, particularly affecting younger animals . Mice deficient in specific inflammatory pathways showed reduced susceptibility to PMA-induced brain injury, suggesting potential therapeutic targets for mitigating its toxic effects .
Table 1: Summary of Biological Activities Associated with PMA
Case Studies
- Zebrafish Model : In a study using zebrafish embryos, PMA exposure resulted in a dose-dependent increase in embryonic deformities and reduced survival rates. The study highlighted the compound's teratogenic effects and its potential mechanisms involving oxidative stress pathways .
- Keratinocyte Response : Human keratinocyte (HaCaT) cells treated with PMA exhibited significant inflammatory responses within hours of treatment. This was characterized by increased cytokine production and cell death over time. The study also explored potential countermeasures using tangeretin, which effectively reduced PMA-induced inflammation .
- Neurodevelopmental Impact : A study on neonatal rodents demonstrated that PMA exposure led to widespread neurodegeneration, particularly affecting younger animals. Mice lacking IL-18 showed protection against these effects, suggesting that targeting inflammatory pathways could mitigate PMA's neurotoxic effects .
Q & A
Basic Research Questions
Q. How should PMA stock solutions be prepared and stored to ensure stability?
PMA is highly lipophilic and requires dissolution in organic solvents. A standard protocol involves preparing a 20 mM stock solution in anhydrous DMSO, vortexing thoroughly, and aliquoting to avoid freeze-thaw cycles. Storage at −20°C in light-protected vials is critical, as PMA degrades under light, moisture, or repeated temperature fluctuations . For aqueous compatibility, dilute stock solutions into buffers containing carriers like β-cyclodextrin (e.g., 20% SBE-β-CD in saline) to maintain solubility .
Q. What is the mechanism of PMA-induced protein kinase C (PKC) activation?
PMA mimics diacylglycerol (DAG) by binding to the C1 domain of PKC isoforms, inducing conformational changes that translocate PKC to the plasma membrane. This activates downstream kinases (e.g., MAPK/ERK) and transcription factors (e.g., NF-κB). Unlike DAG, PMA resists metabolic degradation, causing sustained PKC activation, which is useful for studying long-term effects like cell differentiation or apoptosis .
Q. What negative controls are recommended for PMA experiments?
The 4α-PMA isomer, which lacks PKC-activating properties due to stereochemical differences, serves as a critical negative control. For example, 4α-PMA (5 mg/mL in DMSO) can rule out off-target effects in PKC signaling assays . Solvent controls (e.g., DMSO at equivalent concentrations) are also essential to account for vehicle-induced artifacts .
Advanced Research Questions
Q. How can contradictory results in PMA-mediated cellular responses (e.g., proliferation vs. apoptosis) be resolved?
Discrepancies often arise from concentration-dependent effects. Low PMA doses (1–10 nM) typically promote proliferation via PKC-α/β, while higher doses (>100 nM) induce apoptosis through PKC-δ/θ or reactive oxygen species (ROS). Validate dose-response curves using viability assays (e.g., MTT) and confirm PKC isoform expression in your cell model. For example, THP-1 monocytes differentiate into macrophages at 100 nM PMA over 48–72 hours, but prolonged exposure may trigger cell death .
Q. What experimental considerations are critical for time-course studies with PMA?
- Pulse vs. chronic exposure: Short-term PMA treatment (minutes) examines acute signaling (e.g., ERK phosphorylation), while chronic exposure (days) assesses differentiation or senescence.
- Solvent compatibility: Avoid solvents like ethanol in long-term cultures due to cytotoxicity; use β-cyclodextrin formulations instead .
- Batch variability: Validate PMA activity across batches using TLC (≥98% purity) and functional assays (e.g., NF-κB luciferase reporters) .
Q. How can PMA’s solvent interactions confound results in multi-agent studies?
PMA’s solubility in non-polar solvents (e.g., corn oil) may limit compatibility with hydrophilic drugs. Pre-test solvent interactions using vehicle controls. For example, co-administering PMA with water-soluble inhibitors (e.g., PKC inhibitors) requires phase separation checks via dynamic light scattering .
Q. What strategies mitigate PMA’s photolability and batch-dependent variability?
- Photoprotection: Use amber vials and minimize light exposure during handling .
- Bioactivity validation: Test each batch in a standardized assay (e.g., PKC translocation via immunofluorescence) .
- Structural confirmation: Employ ESI-FT-ICR-MS or NMR to verify acyl group integrity, as ester hydrolysis can reduce potency .
Q. Methodological Resources
- Purity Analysis: TLC (Silica gel 60 F254, chloroform:methanol 9:1) to confirm ≥98% purity .
- Functional Assays: Western blot for PKC phosphorylation (e.g., p-PKCα/βII Thr638/641) or qPCR for differentiation markers (e.g., CD11b in THP-1 cells) .
- Safety Protocols: Use nitrile gloves, chemical-resistant suits, and fume hoods to handle PMA, given its acute toxicity (H300, H310, H330) .
Propriétés
IUPAC Name |
[(1S,2S,6R,10S,11S,13S,14R,15R)-1,6,14-trihydroxy-8-(hydroxymethyl)-4,12,12,15-tetramethyl-5-oxo-13-tetracyclo[8.5.0.02,6.011,13]pentadeca-3,8-dienyl] tetradecanoate | |
---|---|---|
Source | PubChem | |
URL | https://pubchem.ncbi.nlm.nih.gov | |
Description | Data deposited in or computed by PubChem | |
InChI |
InChI=1S/C34H54O7/c1-6-7-8-9-10-11-12-13-14-15-16-17-27(36)41-34-28(31(34,4)5)25-19-24(21-35)20-32(39)26(18-22(2)29(32)37)33(25,40)23(3)30(34)38/h18-19,23,25-26,28,30,35,38-40H,6-17,20-21H2,1-5H3/t23-,25+,26-,28+,30-,32-,33-,34-/m1/s1 | |
Source | PubChem | |
URL | https://pubchem.ncbi.nlm.nih.gov | |
Description | Data deposited in or computed by PubChem | |
InChI Key |
CESGKXMBHGUQTB-JWHXDPQNSA-N | |
Source | PubChem | |
URL | https://pubchem.ncbi.nlm.nih.gov | |
Description | Data deposited in or computed by PubChem | |
Canonical SMILES |
CCCCCCCCCCCCCC(=O)OC12C(C1(C)C)C3C=C(CC4(C(C3(C(C2O)C)O)C=C(C4=O)C)O)CO | |
Source | PubChem | |
URL | https://pubchem.ncbi.nlm.nih.gov | |
Description | Data deposited in or computed by PubChem | |
Isomeric SMILES |
CCCCCCCCCCCCCC(=O)O[C@@]12[C@H](C1(C)C)[C@@H]3C=C(C[C@]4([C@H]([C@]3([C@@H]([C@H]2O)C)O)C=C(C4=O)C)O)CO | |
Source | PubChem | |
URL | https://pubchem.ncbi.nlm.nih.gov | |
Description | Data deposited in or computed by PubChem | |
Molecular Formula |
C34H54O7 | |
Source | PubChem | |
URL | https://pubchem.ncbi.nlm.nih.gov | |
Description | Data deposited in or computed by PubChem | |
DSSTOX Substance ID |
DTXSID10648432 | |
Record name | (1aS,1bS,4aR,7aS,7bS,8R,9R,9aS)-4a,7b,9-Trihydroxy-3-(hydroxymethyl)-1,1,6,8-tetramethyl-5-oxo-1,1a,1b,4,4a,5,7a,7b,8,9-decahydro-9aH-cyclopropa[3,4]benzo[1,2-e]azulen-9a-yl tetradecanoate | |
Source | EPA DSSTox | |
URL | https://comptox.epa.gov/dashboard/DTXSID10648432 | |
Description | DSSTox provides a high quality public chemistry resource for supporting improved predictive toxicology. | |
Molecular Weight |
574.8 g/mol | |
Source | PubChem | |
URL | https://pubchem.ncbi.nlm.nih.gov | |
Description | Data deposited in or computed by PubChem | |
CAS No. |
115905-51-6 | |
Record name | (1aS,1bS,4aR,7aS,7bS,8R,9R,9aS)-4a,7b,9-Trihydroxy-3-(hydroxymethyl)-1,1,6,8-tetramethyl-5-oxo-1,1a,1b,4,4a,5,7a,7b,8,9-decahydro-9aH-cyclopropa[3,4]benzo[1,2-e]azulen-9a-yl tetradecanoate | |
Source | EPA DSSTox | |
URL | https://comptox.epa.gov/dashboard/DTXSID10648432 | |
Description | DSSTox provides a high quality public chemistry resource for supporting improved predictive toxicology. | |
Avertissement et informations sur les produits de recherche in vitro
Veuillez noter que tous les articles et informations sur les produits présentés sur BenchChem sont destinés uniquement à des fins informatives. Les produits disponibles à l'achat sur BenchChem sont spécifiquement conçus pour des études in vitro, qui sont réalisées en dehors des organismes vivants. Les études in vitro, dérivées du terme latin "in verre", impliquent des expériences réalisées dans des environnements de laboratoire contrôlés à l'aide de cellules ou de tissus. Il est important de noter que ces produits ne sont pas classés comme médicaments et n'ont pas reçu l'approbation de la FDA pour la prévention, le traitement ou la guérison de toute condition médicale, affection ou maladie. Nous devons souligner que toute forme d'introduction corporelle de ces produits chez les humains ou les animaux est strictement interdite par la loi. Il est essentiel de respecter ces directives pour assurer la conformité aux normes légales et éthiques en matière de recherche et d'expérimentation.