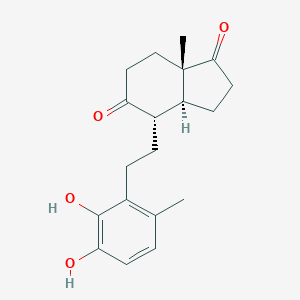
3,4-Dihydroxy-9,10-secoandrosta-1,3,5(10)-triene-9,17-dione
Vue d'ensemble
Description
3,4-dihydroxy-9,10-secoandrosta-1(10),2,4-triene-9,17-dione is an organic compound belonging to the class of catechols, which are compounds containing a 1,2-benzenediol moiety . This compound is an intermediate product in the metabolism of cholesterol by Mycobacterium tuberculosis, the bacteria responsible for tuberculosis .
Applications De Recherche Scientifique
This compound has several scientific research applications. In chemistry, it is used as a model compound to study the metabolism of steroids and the action of dioxygenases . In biology and medicine, it is studied for its role in the metabolism of cholesterol and its potential implications in tuberculosis research . Industrially, it is used in biotransformation processes to produce steroid derivatives .
Mécanisme D'action
The mechanism of action of 3,4-dihydroxy-9,10-secoandrosta-1(10),2,4-triene-9,17-dione involves its interaction with iron-dependent extradiol dioxygenases . These enzymes catalyze the cleavage of the aromatic ring, leading to the formation of various metabolites. The molecular targets include specific enzymes involved in steroid metabolism .
Méthodes De Préparation
The synthesis of 3,4-dihydroxy-9,10-secoandrosta-1(10),2,4-triene-9,17-dione involves several steps. One common method includes the oxidation of cholesterol derivatives. The reaction conditions typically involve the use of specific enzymes or chemical reagents that facilitate the cleavage of the steroid ring structure . Industrial production methods may involve biotransformation processes using bacterial cultures that express the necessary enzymes .
Analyse Des Réactions Chimiques
3,4-dihydroxy-9,10-secoandrosta-1(10),2,4-triene-9,17-dione undergoes various chemical reactions, including oxidation, reduction, and substitution reactions. Common reagents used in these reactions include oxygen, hydrogen, and various catalysts . Major products formed from these reactions include 3-hydroxy-5,9,17-trioxo-4,5:9,10-disecoandrosta-1(10),2-diene-4-oic acid .
Comparaison Avec Des Composés Similaires
Similar compounds to 3,4-dihydroxy-9,10-secoandrosta-1(10),2,4-triene-9,17-dione include other catechols and steroid derivatives. Some examples are 3,4-dihydroxy-9,10-secoandrosta-1,3,5(10)-triene-9,17-dione and 3-hydroxy-5,9,17-trioxo-4,5:9,10-disecoandrosta-1(10),2-diene-4-oic acid . The uniqueness of 3,4-dihydroxy-9,10-secoandrosta-1(10),2,4-triene-9,17-dione lies in its specific structure and its role as an intermediate in cholesterol metabolism by Mycobacterium tuberculosis .
Propriétés
IUPAC Name |
(3aS,4S,7aS)-4-[2-(2,3-dihydroxy-6-methylphenyl)ethyl]-7a-methyl-2,3,3a,4,6,7-hexahydroindene-1,5-dione | |
---|---|---|
Source | PubChem | |
URL | https://pubchem.ncbi.nlm.nih.gov | |
Description | Data deposited in or computed by PubChem | |
InChI |
InChI=1S/C19H24O4/c1-11-3-7-16(21)18(23)12(11)4-5-13-14-6-8-17(22)19(14,2)10-9-15(13)20/h3,7,13-14,21,23H,4-6,8-10H2,1-2H3/t13-,14-,19-/m0/s1 | |
Source | PubChem | |
URL | https://pubchem.ncbi.nlm.nih.gov | |
Description | Data deposited in or computed by PubChem | |
InChI Key |
YUHVBHDSVLKFNI-NJSLBKSFSA-N | |
Source | PubChem | |
URL | https://pubchem.ncbi.nlm.nih.gov | |
Description | Data deposited in or computed by PubChem | |
Canonical SMILES |
CC1=C(C(=C(C=C1)O)O)CCC2C3CCC(=O)C3(CCC2=O)C | |
Source | PubChem | |
URL | https://pubchem.ncbi.nlm.nih.gov | |
Description | Data deposited in or computed by PubChem | |
Isomeric SMILES |
CC1=C(C(=C(C=C1)O)O)CC[C@H]2[C@@H]3CCC(=O)[C@]3(CCC2=O)C | |
Source | PubChem | |
URL | https://pubchem.ncbi.nlm.nih.gov | |
Description | Data deposited in or computed by PubChem | |
Molecular Formula |
C19H24O4 | |
Source | PubChem | |
URL | https://pubchem.ncbi.nlm.nih.gov | |
Description | Data deposited in or computed by PubChem | |
DSSTOX Substance ID |
DTXSID701028828 | |
Record name | 3,4-Dihydroxy-9,10-secoandrosta-1,3,5(10)-triene-9,17-dione | |
Source | EPA DSSTox | |
URL | https://comptox.epa.gov/dashboard/DTXSID701028828 | |
Description | DSSTox provides a high quality public chemistry resource for supporting improved predictive toxicology. | |
Molecular Weight |
316.4 g/mol | |
Source | PubChem | |
URL | https://pubchem.ncbi.nlm.nih.gov | |
Description | Data deposited in or computed by PubChem | |
CAS RN |
2168-61-8 | |
Record name | 3,4-Dihydroxy-9,10-secoandrosta-1,3,5(10)-triene-9,17-dione | |
Source | EPA DSSTox | |
URL | https://comptox.epa.gov/dashboard/DTXSID701028828 | |
Description | DSSTox provides a high quality public chemistry resource for supporting improved predictive toxicology. | |
Retrosynthesis Analysis
AI-Powered Synthesis Planning: Our tool employs the Template_relevance Pistachio, Template_relevance Bkms_metabolic, Template_relevance Pistachio_ringbreaker, Template_relevance Reaxys, Template_relevance Reaxys_biocatalysis model, leveraging a vast database of chemical reactions to predict feasible synthetic routes.
One-Step Synthesis Focus: Specifically designed for one-step synthesis, it provides concise and direct routes for your target compounds, streamlining the synthesis process.
Accurate Predictions: Utilizing the extensive PISTACHIO, BKMS_METABOLIC, PISTACHIO_RINGBREAKER, REAXYS, REAXYS_BIOCATALYSIS database, our tool offers high-accuracy predictions, reflecting the latest in chemical research and data.
Strategy Settings
Precursor scoring | Relevance Heuristic |
---|---|
Min. plausibility | 0.01 |
Model | Template_relevance |
Template Set | Pistachio/Bkms_metabolic/Pistachio_ringbreaker/Reaxys/Reaxys_biocatalysis |
Top-N result to add to graph | 6 |
Feasible Synthetic Routes
Q & A
Q1: What is the significance of 3,4-DHSA in microbial steroid degradation?
A: 3,4-DHSA is a key intermediate in the degradation of cholesterol and other steroids by certain microorganisms [, , , ]. This compound is formed during the breakdown of the steroid A ring, a crucial step in utilizing these complex molecules as a carbon source.
Q2: What enzyme is responsible for the formation of 3,4-DHSA?
A: Research suggests that 3,4-DHSA is formed from the precursor 3-hydroxy-9,10-secoandrosta-1,3,5(10)-triene-9,17-dione []. The enzyme responsible for this transformation has not been definitively identified, but studies indicate it likely involves a dioxygenase mechanism [, ].
Q3: How is 3,4-DHSA further metabolized by microorganisms?
A: 3,4-DHSA is further degraded by the enzyme 3,4-dihydroxy-9,10-secoandrosta-1,3,5(10)-triene-9,17-dione 4,5-dioxygenase (4,5-dioxygenase) [, , ]. This enzyme catalyzes the oxygenolytic cleavage of the steroid ring A, leading to the formation of 4(5),9(10)-diseco-3-hydroxyandrosta-1(10),2-diene-5,9,17-trion-4-oic acid []. Subsequent metabolic steps ultimately result in the formation of smaller molecules like 2-oxo-4-hydroxycaproic acid and 3aα-H-4α-[3'-propionic acid]-7aβ-methylhexahydro-1,5-indanedione, which can be utilized by the microorganism [].
Q4: Why is the metabolism of 3,4-DHSA relevant to Mycobacterium tuberculosis?
A: Research indicates that M. tuberculosis possesses a gene cluster similar to that found in Rhodococcus sp. strain RHA1, which is involved in cholesterol degradation []. This cluster includes the hsaC gene, suggesting that M. tuberculosis can also catabolize cholesterol via 3,4-DHSA []. This metabolic capability may be crucial for the survival of M. tuberculosis within macrophages, highlighting its potential as a target for novel therapeutics [].
Q5: What are the kinetic characteristics of the 3,4-DHSA 4,5-dioxygenase enzyme?
A: Kinetic studies of the 3,4-DHSA 4,5-dioxygenase indicate that it follows an ordered Bi-Uni mechanism, with molecular oxygen binding first, followed by 3,4-DHSA []. The enzyme then releases the product, 4(5),9(10)-diseco-3-hydroxyandrosta-1(10),2-diene-5,9,17-trion-4-oic acid. Interestingly, the kinetic data also suggest the formation of a dead-end complex involving the enzyme, oxygen, and the product [].
Avertissement et informations sur les produits de recherche in vitro
Veuillez noter que tous les articles et informations sur les produits présentés sur BenchChem sont destinés uniquement à des fins informatives. Les produits disponibles à l'achat sur BenchChem sont spécifiquement conçus pour des études in vitro, qui sont réalisées en dehors des organismes vivants. Les études in vitro, dérivées du terme latin "in verre", impliquent des expériences réalisées dans des environnements de laboratoire contrôlés à l'aide de cellules ou de tissus. Il est important de noter que ces produits ne sont pas classés comme médicaments et n'ont pas reçu l'approbation de la FDA pour la prévention, le traitement ou la guérison de toute condition médicale, affection ou maladie. Nous devons souligner que toute forme d'introduction corporelle de ces produits chez les humains ou les animaux est strictement interdite par la loi. Il est essentiel de respecter ces directives pour assurer la conformité aux normes légales et éthiques en matière de recherche et d'expérimentation.