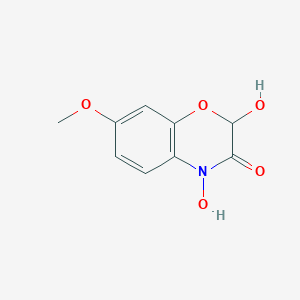
Dimboa
Vue d'ensemble
Description
2,4-Dihydroxy-7-methoxy-2H-1,4-benzoxazin-3(4H)-one (DIMBOA) is a benzoxazinoid (BXD) secondary metabolite predominantly synthesized in maize (Zea mays), wheat (Triticum aestivum), and other Poaceae species. It plays critical roles in plant defense against biotic stressors, including insects, fungi, and bacteria, and exhibits allelopathic properties . This compound is biosynthesized via the shikimate pathway, with its precursor indole-3-glycerol phosphate converted by BENZOXAZINELESS1 (BX1) or INDOLE GLYCEROL PHOSPHATE LYASE (IGL) enzymes under developmental or stress-induced regulation . In planta, this compound exists primarily as its glucoside (this compound-Glc), which stabilizes the compound until hydrolysis releases the bioactive aglucone during stress responses .
Méthodes De Préparation
Natural Extraction Methods from Maize
Enzymatic Release from DIMBOA-Glucoside
This compound exists predominantly as its β-D-glucoside conjugate (this compound-Glc) in plant tissues, requiring enzymatic hydrolysis to release the bioactive aglycone. Homogenization of frozen maize shoots (7-day-old etiolated seedlings) in aqueous medium activates endogenous β-glucosidases, which catalyze the conversion of this compound-Glc to this compound within 1 hour at 20°C . This step is critical for maximizing yield, as incomplete hydrolysis reduces final product purity. Post-homogenization, filtration through cheesecloth removes cellular debris, yielding an aqueous extract rich in this compound .
Solid-Phase Extraction Using Amberlite XAD-7
Amberlite XAD-7, a macroporous adsorbent resin, is widely employed for its high affinity for phenolic compounds like this compound. After enzymatic hydrolysis, the aqueous extract is stirred with Amberlite XAD-7 (10% w/v) for 1 hour, allowing adsorption of this compound onto the resin . Subsequent washing with water removes polar impurities, and elution with acetone recovers the adsorbed this compound. Evaporation of the acetone eluate under reduced pressure yields a crude residue, which is further purified via crystallization in methylene chloride and hexane . This method achieves a purity >98% with a yield of 1.36 ± 0.27 g this compound per kilogram of fresh maize shoots .
Table 1: Large-Scale this compound Isolation Using Amberlite XAD-7
Parameter | Value/Description |
---|---|
Plant Material | 7-day-old etiolated maize seedlings (1 kg) |
Adsorbent | Amberlite XAD-7 (100 g) |
Adsorption Time | 1 hour |
Elution Solvent | Acetone |
Crystallization Solvent | Methylene chloride/hexane |
Yield | 1.36 ± 0.27 g |
Purity | >98% |
Cost-Effective Solvent Extraction Modifications
Challenges with Amberlite XAD-7
While effective, Amberlite XAD-7 is cost-prohibitive for high-throughput applications. Traditional solvent extraction methods using diethyl ether require large volumes (2 L per 150 mg this compound) due to the compound’s water solubility, complicating scalability .
Optimized Ethyl Acetate Partitioning
A modified protocol replaces Amberlite XAD-7 with ethyl acetate partitioning. The aqueous extract (post-enzymatic hydrolysis) is adjusted to pH 3.0 using hydrochloric acid and sequentially extracted with ethyl acetate (3 × 500 mL). The combined organic phases are dried over anhydrous sodium sulfate and concentrated in vacuo, yielding a semi-pure this compound fraction . Further purification via silica gel chromatography (hexane:ethyl acetate, 1:1 v/v) enhances purity to >95%, albeit with a 15% reduction in yield compared to Amberlite-based methods .
Table 2: Comparative Analysis of Extraction Methods
Method | Yield (g/kg) | Purity (%) | Cost (USD/kg) |
---|---|---|---|
Amberlite XAD-7 | 1.36 ± 0.27 | >98 | 120 |
Ethyl Acetate Partition | 1.15 ± 0.18 | >95 | 45 |
Influence of Growth Conditions on this compound Biosynthesis
Light-Mediated Inhibition
This compound accumulation is inversely correlated with light exposure. Etiolated maize seedlings grown in complete darkness for 7 days contain 2.4-fold higher this compound levels (12.7 ± 1.3 mg/g fresh weight) compared to light-exposed counterparts (5.3 ± 0.8 mg/g) . This photoinhibition effect is attributed to light-induced degradation pathways and repression of biosynthetic genes such as Bx1 and Bx2 .
Optimal Harvest Time
This compound concentrations peak in 7-day-old seedlings, declining rapidly thereafter due to enzymatic degradation and oxidative polymerization. Harvesting at 168 hours post-germination ensures maximal yield, with a half-life of <24 hours in aqueous extracts .
Synthetic Approaches and Derivatives
Challenges in Chemical Synthesis
Despite its structural simplicity, this compound synthesis is less economically viable than extraction. Reported routes involve malonamic acid intermediates and cyclization under acidic conditions, but yields remain low (<40%) . For example, N-(2-hydroxy-7-methoxyphenyl)malonamic acid cyclizes in HCl/ethanol to form this compound, requiring multiple purification steps .
Derivatives and Functional Analogues
Efforts to synthesize this compound derivatives, such as BOA (2-benzoxazolinone) and CDHB (2-carboxy-4,7-dimethoxy-1,4-benzoxazin-3-one), highlight the compound’s versatility. These derivatives are typically prepared via O-methylation or hydrolysis of this compound, enabling structure-activity studies against bacterial pathogens like Ralstonia solanacearum .
Analyse Des Réactions Chimiques
Electrophilic Reactions
The open form of benzoxazinones, resulting from oxo-cyclo ring-chain tautomerism, acts as an α-oxo-aldehyde, a potent electrophile. It can react with nucleophilic residues in proteins, such as thiols and amines, potentially causing enzymatic inhibition .
- DIMBOA reacts with butylamine, forming imino products .
- The 2-O-methyl acetal derivative of this compound does not react, suggesting that ring-opening is required for imino adduct formation .
- HMBOA (a lactam) reacts slower with amines than this compound, possibly due to the electron-withdrawing effect of the hydroxamic acid hydroxy group .
- This compound reacts with N-α-acetyl-lysine, a model compound for the ε-amino groups of lysine residues in enzymes .
Degradation
This compound degrades through different pathways, influenced by factors like pH, solvent, and substituents .
- The half-lives of HDMBOA and this compound aglucones are 1.8 and 25 hours, respectively, in buffered D2O at pH 5.5 and 24 °C .
- At pH 8.5 and 48 °C, this compound degrades faster than DIBOA, attributed to the electron-donating effect of the methoxy group at position 7 .
- This compound degradation rates over different pH values show a bell-shaped curve, with a maximum around pH 9.0 .
Two suggested pathways for benzoxazinone degradation are :
- Pathway I: The carbon atom in position 2 is eliminated as formic acid during benzoxazinone degradation. The concentration of hydroxamic acid monoanion, dictated by the pH, is critical to the reaction rate. The hydroxamic acid monoanion acts as an internal nucleophile attacking the aldehyde carbon, forming an isocyanate, ultimately leading to benzoxazolinones. This compound degradation is faster in organic solvents with high donor numbers, which form stronger hydrogen bonds and render the hydroxy group more nucleophilic .
- Pathway II: The phenol function plays an active role in the degradation reaction. The phenol in the open form acts as a nucleophile by attacking the amide carbonyl, producing a 5-membered closed form that is in equilibrium with the other forms. After aldehyde hydration, fragmentation takes place, eliminating formic acid and the N-substituted group. No isocyanate is formed, but rather the less stable tautomer of the benzoxazolinone, which rapidly rearranges yielding the final benzoxazolinone .
Hydrolysis
This compound exists in plants primarily as a glucoside (this compound-Glc) . Upon tissue disruption, β-glucosidases hydrolyze this compound-glc, releasing the biocidal this compound aglycone .
Role of Bx Enzymes
The biosynthesis of this compound-glucoside involves several enzymes :
- BX6: This dioxygenase uses DIBOA-glc as a substrate, producing 2,4,7-trihydroxy-2H-1,4-benzoxazin-3-(4H)-one-glc (TRIBOA-glc) . It exhibits a pH optimum of 6 for the DIBOA-glc hydroxylation reaction. The steady-state kinetic constants for DIBOA-glc and 2-oxoglutarate are shown below :
Enzyme | pH Optima | Substrate | Kinetic Constants (mM) | Vmax (μkat/g) | kcat (s-1) | kcat/Km (mM-1s-1) |
---|---|---|---|---|---|---|
BX6 | 6 | DIBOA-glc | 0.373 ± 0.032 | 59.0 ± 1.5 | 2.1 ± 0.1 | 5.6 ± 1.6 |
- BX7: This O-methyltransferase (OMT) catalyzes the formation of this compound-glc from TRIBOA-glc . It exhibits a pH optimum of 7. The steady-state kinetic constants for TRIBOA-glc are :
Enzyme | pH Optima | Substrate | Kinetic Constants (mM) | Vmax (μkat/g) | kcat (s-1) | kcat/Km (mM-1s-1) |
---|---|---|---|---|---|---|
BX7 | 7 | TRIBOA-glc | 0.272 ± 0.012 | 6.79 ± 0.11 | 0.249 ± 0.004 | 0.91 ± 0.34 |
Other Reactions and Activities
Applications De Recherche Scientifique
Antibacterial and Antifungal Properties
Antibacterial Activity
Research has demonstrated that DIMBOA exhibits significant antibacterial activity against various pathogens. A study evaluated the Minimum Inhibitory Concentration (MIC) and Minimum Bactericidal Concentration (MBC) of this compound against Ralstonia solanacearum, a pathogen affecting tobacco plants. The results indicated that this compound effectively reduced bacterial growth and biofilm formation, highlighting its potential as a biocontrol agent in agriculture .
Antifungal Activity
this compound also shows antifungal properties, particularly in enhancing plant resistance against fungal pathogens. It regulates innate immunity in cereals, contributing to increased resistance against pests like Setosphaeria turtica and aphids .
Insect Resistance
This compound plays a crucial role in plant defense mechanisms against herbivorous insects. Its accumulation in maize is directly correlated with resistance to pests such as the Asian corn borer (Ostrinia nubilalis) and corn leaf aphids. The compound acts as a digestive toxin, reducing the efficiency of food digestion in these insects .
Table 1: Effects of this compound on Insect Resistance
Insect Species | Mode of Action | Reference |
---|---|---|
Asian Corn Borer | Digestive toxin | |
Corn Leaf Aphid | Induces anorexia | |
European Corn Borer | Reduces digestibility |
Allelopathic Effects
This compound is involved in allelopathy, where it influences the growth and development of surrounding plants. Studies have shown that higher concentrations of this compound in root exudates can alter microbial communities in the rhizosphere, promoting beneficial bacteria while suppressing pathogenic species . This property is particularly valuable for sustainable agricultural practices.
Applications in Human Health
Emerging research indicates that this compound may have implications for human health, particularly in cancer research. Preliminary studies suggest that this compound can inhibit the proliferation of mammary epithelial cells, indicating potential anti-cancer properties . Further investigations are needed to explore its therapeutic applications.
Genetic Variation and Accumulation
The accumulation of this compound is influenced by genetic factors within maize species. Research has shown that certain genotypes exhibit higher levels of this compound, correlating with enhanced resistance to insect pests . Understanding these genetic variations can aid in breeding programs aimed at developing pest-resistant crops.
Case Studies
Case Study 1: Tobacco Bacterial Wilt Control
In greenhouse experiments, this compound was applied to tobacco seedlings infected with R. solanacearum. The treatment significantly reduced the disease index, demonstrating its efficacy as a natural pesticide .
Case Study 2: Maize-Derived Exudates
Field studies assessed the impact of natural variations in this compound concentrations on maize's rhizosphere microbiome. Results indicated that higher this compound levels enriched metabolic pathways associated with plant disease resistance, showcasing its role in sustainable farming practices .
Mécanisme D'action
DIMBOA exerts its effects primarily through its toxicity to pests and pathogens. It inhibits the biosynthesis and accumulation of toxic trichothecenes by affecting the expression of genes such as Tri6 and Tri5 . This compound also exhibits cytotoxicity to plant cells, causing plasmolysis, cell collapse, and cell rupture . Additionally, this compound functions as a signaling molecule, leading to the accumulation of callose in response to fungal elicitors and insect feeding .
Comparaison Avec Des Composés Similaires
Structural and Functional Comparison with Analogous Compounds
DIMBOA shares structural and functional similarities with other benzoxazinoids and degradation products. Key comparisons are outlined below:
Structural Analogues
DIBOA (2,4-Dihydroxy-2H-1,4-benzoxazin-3(4H)-one)
- Structural Difference : Lacks the 7-methoxy group present in this compound.
- Biosynthesis : Precursor to this compound in wheat; chromosome 4B in hexaploid wheat regulates its conversion to this compound .
- Bioactivity : Less stable than this compound, with weaker antifungal and anti-insect activity .
- Accumulation : Nullisomic-tetrasomic wheat lines (e.g., CS-N4AT4B) show minimal DIBOA accumulation but high this compound levels, indicating metabolic prioritization toward this compound .
MBOA (6-Methoxy-2-benzoxazolinone)
- Structural Difference : Degradation product of this compound via spontaneous cyclization under alkaline conditions.
- Bioactivity : Reduced toxicity compared to this compound; primarily detoxified by insects via glucosylation (MBOA-Glc) .
- Metabolism : In Spodoptera frugiperda, 13% of ingested this compound is converted to MBOA, which is further glucosylated to MBOA-Glc for excretion .
HMBOA (2-Hydroxy-4-methoxy-1,4-benzoxazin-3-one)
- Structural Difference : Reduced lactam form of this compound.
- Bioactivity : Less toxic than this compound; produced by insect UGT enzymes (e.g., SfUGT33F28/40L8) as a detoxification strategy .
- Metabolism : Constitutes ~44% of this compound metabolites in S. frugiperda frass .
Functional Analogues
HDMBOA-Glc (2-Hydroxy-4,7-dimethoxy-1,4-benzoxazin-3-one-glucoside)
- Role : Induced during fungal infections (e.g., Setosphaeria turcica) and acts synergistically with this compound to enhance apoplastic defense .
- Regulation : Accumulates in maize apoplast during later infection stages, unlike this compound-Glc, which peaks earlier .
BOA (Benzoxazolin-2-one)
- Role : Degradation product of DIBOA; exhibits allelopathic activity but lower antimicrobial efficacy than this compound .
Comparative Bioactivity and Metabolic Pathways
Antimicrobial and Insecticidal Activity
Induction and Accumulation Dynamics
- This compound: Induced by elicitors (e.g., chitosan, herbivore-associated molecular patterns) in maize roots and shoots . Levels decline in Bt maize compared to non-transgenic lines, reducing pest resistance .
- This compound-Glc :
- HDMBOA-Glc :
- Sustained accumulation in maize apoplast during prolonged fungal infection .
Activité Biologique
Introduction
DIMBOA (2,4-dihydroxy-7-methoxy-1,4-benzoxazin-3-one) is a naturally occurring benzoxazinoid compound predominantly found in maize (Zea mays). It plays a significant role in plant defense mechanisms, exhibiting various biological activities including antimicrobial, insecticidal, and allelopathic properties. This article provides a detailed overview of the biological activity of this compound, supported by data tables, case studies, and research findings.
1. Antimicrobial Activity
This compound has demonstrated considerable antibacterial and antifungal properties. Research has shown that it inhibits the growth of Ralstonia solanacearum, a pathogen responsible for bacterial wilt in plants. The minimum inhibitory concentration (MIC) and minimum bactericidal concentration (MBC) of this compound against this pathogen were found to be 58.55 mg/L and 208.92 mg/L, respectively .
Table 1: Antimicrobial Activity of this compound
Pathogen | MIC (mg/L) | MBC (mg/L) |
---|---|---|
Ralstonia solanacearum | 58.55 | 208.92 |
Other tested bacteria | Varies | Varies |
2. Insecticidal Activity
This compound serves as an effective biocidal agent against various insect pests, including aphids and caterpillars such as Spodoptera littoralis. The compound induces physiological changes in insects that lead to reduced feeding and increased mortality rates .
Case Study: Impact on Spodoptera littoralis
In a study examining the effects of this compound on the fourth larval instar of Spodoptera littoralis, it was observed that treatment with this compound significantly decreased the total hemocyte count (THC) and induced ultrastructural alterations in hemocytes, suggesting a compromised immune response .
3. Role in Plant Defense Mechanisms
This compound is crucial for maize's innate immunity against herbivory and pathogen attack. It acts as a signaling molecule that regulates defense gene expression and promotes callose deposition in response to pathogen-associated molecular patterns (PAMPs) . This regulatory function enhances the plant's resistance to pests and diseases.
Table 2: this compound's Role in Plant Defense
Function | Mechanism |
---|---|
Defense signaling | Regulates gene expression for defense responses |
Callose deposition | Enhances structural barriers against pathogens |
Induction of insect resistance | Rapid accumulation upon insect feeding |
4. Influence on Soil Microbiome
Recent studies have highlighted the impact of this compound on soil microbial communities through root exudation. Higher concentrations of this compound in root exudates were shown to enrich specific metabolic pathways associated with plant disease resistance, thereby influencing the rhizosphere microbiome composition .
Table 3: Effects of this compound on Soil Microbiome
Parameter | Effect |
---|---|
Root exudation levels | Increased microbial diversity |
Metabolic pathways enriched | Disease resistance pathways |
Q & A
Basic Research Questions
Q. What standardized methods are recommended for isolating and purifying DIMBOA from plant tissues?
this compound is typically isolated from etiolated maize seedlings using solvent extraction followed by chromatographic purification. Key steps include methanol extraction, centrifugation, and RP-HPLC analysis. Structural validation employs ¹H NMR (δ 3.31 ppm in CD₃OD) and melting point determination (168–169°C) . For comparative studies, this compound and its analog DIBOA (isolated from rye) require species-specific protocols to avoid cross-contamination.
Q. How can researchers quantify this compound in plant tissues, and what are common pitfalls?
Quantification via RP-HPLC with UV detection is standard. Calibration curves using purified this compound standards are essential. Pitfalls include degradation during extraction (this compound is heat- and light-sensitive) and interference from glucosylated derivatives like this compound-Glc. Validation via spiked recovery experiments is recommended to ensure accuracy .
Q. What is the role of this compound in plant defense mechanisms against biotic stressors?
this compound acts as a phytoanticipin, deterring aphids (Rhopalosiphum maidis) and fungi via direct toxicity and indirect mechanisms like callose induction. Aphid resistance correlates with this compound content (r = –0.361, P < 0.01), but its efficacy depends on genetic background (e.g., Bx1 gene functionality) and interactions with catabolic products like HDMBOA-Glc .
Advanced Research Questions
Q. How do genetic loci regulate this compound biosynthesis, and what experimental approaches resolve contradictory findings?
QTL mapping in maize IBM populations identified chromosome 4 (near Bx1) and chromosome 5 as critical for this compound-Glc accumulation. However, aphid resistance in near-isogenic lines (NILs) with introgressed B73 QTLs showed reduced this compound levels but retained resistance, implicating epistatic interactions or non-Bx pathways. Combinatorial approaches (e.g., transposon mutagenesis in Bx1::Ds lines, transcriptomics) are needed to dissect these paradoxes .
Q. What methodologies differentiate this compound’s direct antimicrobial effects from its role in priming plant immune responses?
Dual assays are required:
- In vitro bioassays (e.g., fungal growth inhibition by MBOA vs. This compound degradation by Ustilago maydis ).
- In planta studies tracking this compound translocation (e.g., shoot-to-root movement under high-density stress ).
- Transcriptional profiling of defense genes (e.g., jasmonate pathway markers) in this compound-deficient mutants.
Q. How can researchers address contradictions between this compound’s phytotoxic and protective roles in herbicide interactions?
XAFS and Fenton assays reveal this compound chelates Fe³⁺, mitigating oxidative stress from chiral herbicides. Experimental designs must control for Fe homeostasis (e.g., iron-limited growth media) and use isotopic labeling to track this compound-Fe complexes .
Q. Methodological Considerations
Q. What statistical approaches are optimal for analyzing this compound-related QTL data with low heritability?
- Multi-environment QTL mapping to account for genotype × environment interactions.
- Partial least squares regression (PLSR) to integrate metabolite (this compound, HDMBOA-Glc) and phenotypic (aphid fecundity, callose spots/mm²) data .
- Bayesian networks to model epistasis between Bx genes and resistance loci on chromosome 6 .
Q. How should researchers validate this compound’s role in callose induction when genetic redundancy exists?
Combine:
- Bx1 knockouts (e.g., Bx1::Ds lines) to abolish this compound production .
- Pharmacological inhibition of callose synthase (e.g., dexamethasone treatments).
- Confocal microscopy to quantify aphid-induced callose in vascular bundles .
Q. Data Contradiction Management
Q. How to reconcile studies where low-DIMBOA lines exhibit aphid resistance?
Investigate compensatory mechanisms:
- Screen for alternative benzoxazinoids (e.g., HDMBOA-Glc) via LC-MS/MS.
- Evaluate non-Bx resistance factors (e.g., silica deposition, volatile organic compounds) in NILs .
Q. What controls are essential when studying this compound degradation by microbial pathogens?
Include:
- Heat-killed pathogen controls to distinguish enzymatic vs. spontaneous degradation.
- pH-adjusted media to mimic apoplastic conditions.
- Quantification of degradation products (e.g., MBOA) via GC-MS .
Propriétés
IUPAC Name |
2,4-dihydroxy-7-methoxy-1,4-benzoxazin-3-one | |
---|---|---|
Source | PubChem | |
URL | https://pubchem.ncbi.nlm.nih.gov | |
Description | Data deposited in or computed by PubChem | |
InChI |
InChI=1S/C9H9NO5/c1-14-5-2-3-6-7(4-5)15-9(12)8(11)10(6)13/h2-4,9,12-13H,1H3 | |
Source | PubChem | |
URL | https://pubchem.ncbi.nlm.nih.gov | |
Description | Data deposited in or computed by PubChem | |
InChI Key |
GDNZNIJPBQATCZ-UHFFFAOYSA-N | |
Source | PubChem | |
URL | https://pubchem.ncbi.nlm.nih.gov | |
Description | Data deposited in or computed by PubChem | |
Canonical SMILES |
COC1=CC2=C(C=C1)N(C(=O)C(O2)O)O | |
Source | PubChem | |
URL | https://pubchem.ncbi.nlm.nih.gov | |
Description | Data deposited in or computed by PubChem | |
Molecular Formula |
C9H9NO5 | |
Source | PubChem | |
URL | https://pubchem.ncbi.nlm.nih.gov | |
Description | Data deposited in or computed by PubChem | |
DSSTOX Substance ID |
DTXSID90936021 | |
Record name | 2,4-Dihydroxy-7-methoxy-1,4-benzoxazin-3-one | |
Source | EPA DSSTox | |
URL | https://comptox.epa.gov/dashboard/DTXSID90936021 | |
Description | DSSTox provides a high quality public chemistry resource for supporting improved predictive toxicology. | |
Molecular Weight |
211.17 g/mol | |
Source | PubChem | |
URL | https://pubchem.ncbi.nlm.nih.gov | |
Description | Data deposited in or computed by PubChem | |
Physical Description |
Solid | |
Record name | 2,4-Dihydroxy-7-methoxy-2H-1,4-benzoxazin-3(4H)-one | |
Source | Human Metabolome Database (HMDB) | |
URL | http://www.hmdb.ca/metabolites/HMDB0034864 | |
Description | The Human Metabolome Database (HMDB) is a freely available electronic database containing detailed information about small molecule metabolites found in the human body. | |
Explanation | HMDB is offered to the public as a freely available resource. Use and re-distribution of the data, in whole or in part, for commercial purposes requires explicit permission of the authors and explicit acknowledgment of the source material (HMDB) and the original publication (see the HMDB citing page). We ask that users who download significant portions of the database cite the HMDB paper in any resulting publications. | |
CAS No. |
15893-52-4 | |
Record name | 2,4-Dihydroxy-7-methoxy-1,4-benzoxazin-3-one | |
Source | CAS Common Chemistry | |
URL | https://commonchemistry.cas.org/detail?cas_rn=15893-52-4 | |
Description | CAS Common Chemistry is an open community resource for accessing chemical information. Nearly 500,000 chemical substances from CAS REGISTRY cover areas of community interest, including common and frequently regulated chemicals, and those relevant to high school and undergraduate chemistry classes. This chemical information, curated by our expert scientists, is provided in alignment with our mission as a division of the American Chemical Society. | |
Explanation | The data from CAS Common Chemistry is provided under a CC-BY-NC 4.0 license, unless otherwise stated. | |
Record name | 2,4-Dihydroxy-7-methoxy-1,4-benzoxazin-3-one | |
Source | ChemIDplus | |
URL | https://pubchem.ncbi.nlm.nih.gov/substance/?source=chemidplus&sourceid=0015893524 | |
Description | ChemIDplus is a free, web search system that provides access to the structure and nomenclature authority files used for the identification of chemical substances cited in National Library of Medicine (NLM) databases, including the TOXNET system. | |
Record name | 2,4-Dihydroxy-7-methoxy-1,4-benzoxazin-3-one | |
Source | EPA DSSTox | |
URL | https://comptox.epa.gov/dashboard/DTXSID90936021 | |
Description | DSSTox provides a high quality public chemistry resource for supporting improved predictive toxicology. | |
Record name | 2,4-DIHYDROXY-7-METHOXY-1,4-BENZOXAZIN-3-ONE | |
Source | FDA Global Substance Registration System (GSRS) | |
URL | https://gsrs.ncats.nih.gov/ginas/app/beta/substances/TI783RU0DR | |
Description | The FDA Global Substance Registration System (GSRS) enables the efficient and accurate exchange of information on what substances are in regulated products. Instead of relying on names, which vary across regulatory domains, countries, and regions, the GSRS knowledge base makes it possible for substances to be defined by standardized, scientific descriptions. | |
Explanation | Unless otherwise noted, the contents of the FDA website (www.fda.gov), both text and graphics, are not copyrighted. They are in the public domain and may be republished, reprinted and otherwise used freely by anyone without the need to obtain permission from FDA. Credit to the U.S. Food and Drug Administration as the source is appreciated but not required. | |
Record name | 2,4-Dihydroxy-7-methoxy-2H-1,4-benzoxazin-3(4H)-one | |
Source | Human Metabolome Database (HMDB) | |
URL | http://www.hmdb.ca/metabolites/HMDB0034864 | |
Description | The Human Metabolome Database (HMDB) is a freely available electronic database containing detailed information about small molecule metabolites found in the human body. | |
Explanation | HMDB is offered to the public as a freely available resource. Use and re-distribution of the data, in whole or in part, for commercial purposes requires explicit permission of the authors and explicit acknowledgment of the source material (HMDB) and the original publication (see the HMDB citing page). We ask that users who download significant portions of the database cite the HMDB paper in any resulting publications. | |
Melting Point |
168 - 169 °C | |
Record name | 2,4-Dihydroxy-7-methoxy-2H-1,4-benzoxazin-3(4H)-one | |
Source | Human Metabolome Database (HMDB) | |
URL | http://www.hmdb.ca/metabolites/HMDB0034864 | |
Description | The Human Metabolome Database (HMDB) is a freely available electronic database containing detailed information about small molecule metabolites found in the human body. | |
Explanation | HMDB is offered to the public as a freely available resource. Use and re-distribution of the data, in whole or in part, for commercial purposes requires explicit permission of the authors and explicit acknowledgment of the source material (HMDB) and the original publication (see the HMDB citing page). We ask that users who download significant portions of the database cite the HMDB paper in any resulting publications. | |
Retrosynthesis Analysis
AI-Powered Synthesis Planning: Our tool employs the Template_relevance Pistachio, Template_relevance Bkms_metabolic, Template_relevance Pistachio_ringbreaker, Template_relevance Reaxys, Template_relevance Reaxys_biocatalysis model, leveraging a vast database of chemical reactions to predict feasible synthetic routes.
One-Step Synthesis Focus: Specifically designed for one-step synthesis, it provides concise and direct routes for your target compounds, streamlining the synthesis process.
Accurate Predictions: Utilizing the extensive PISTACHIO, BKMS_METABOLIC, PISTACHIO_RINGBREAKER, REAXYS, REAXYS_BIOCATALYSIS database, our tool offers high-accuracy predictions, reflecting the latest in chemical research and data.
Strategy Settings
Precursor scoring | Relevance Heuristic |
---|---|
Min. plausibility | 0.01 |
Model | Template_relevance |
Template Set | Pistachio/Bkms_metabolic/Pistachio_ringbreaker/Reaxys/Reaxys_biocatalysis |
Top-N result to add to graph | 6 |
Feasible Synthetic Routes
Avertissement et informations sur les produits de recherche in vitro
Veuillez noter que tous les articles et informations sur les produits présentés sur BenchChem sont destinés uniquement à des fins informatives. Les produits disponibles à l'achat sur BenchChem sont spécifiquement conçus pour des études in vitro, qui sont réalisées en dehors des organismes vivants. Les études in vitro, dérivées du terme latin "in verre", impliquent des expériences réalisées dans des environnements de laboratoire contrôlés à l'aide de cellules ou de tissus. Il est important de noter que ces produits ne sont pas classés comme médicaments et n'ont pas reçu l'approbation de la FDA pour la prévention, le traitement ou la guérison de toute condition médicale, affection ou maladie. Nous devons souligner que toute forme d'introduction corporelle de ces produits chez les humains ou les animaux est strictement interdite par la loi. Il est essentiel de respecter ces directives pour assurer la conformité aux normes légales et éthiques en matière de recherche et d'expérimentation.