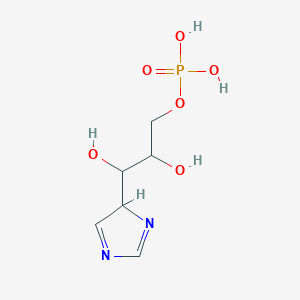
D-érythro-Imidazoleglycérol Phosphate Monohydraté
Vue d'ensemble
Description
D-erythro-Imidazoleglycerol Phosphate Monohydrate: is a biochemical reagent with the molecular formula C6H13N2O7P and a molecular weight of 256.15 g/mol . This compound is a crucial intermediate in the biosynthesis of histidine, an essential amino acid. It features a unique molecular structure that includes an imidazole ring, a glycerol backbone, and a phosphate group .
Applications De Recherche Scientifique
D-erythro-Imidazoleglycerol Phosphate Monohydrate has several scientific research applications, including:
Mécanisme D'action
Target of Action
D-erythro-Imidazoleglycerol Phosphate Monohydrate is a specialized biochemical compound that primarily targets enzymes involved in the biosynthesis of histidine . The compound’s unique molecular structure, which includes an imidazole ring, a glycerol backbone, and a phosphate group, allows it to interact with these enzymes .
Mode of Action
The compound acts as a substrate for these enzymes, participating in the catalytic reactions they facilitate
Biochemical Pathways
D-erythro-Imidazoleglycerol Phosphate Monohydrate plays a crucial role in the histidine biosynthesis pathway . It can be synthesized from D-ribose 5-phosphate and formamidine . The compound’s involvement in this pathway affects the production of histidine, an essential amino acid.
Result of Action
The action of D-erythro-Imidazoleglycerol Phosphate Monohydrate in the histidine biosynthesis pathway contributes to the production of histidine . As an essential amino acid, histidine plays a vital role in protein synthesis and other biological functions. Therefore, the compound’s action has significant molecular and cellular effects.
Analyse Biochimique
Biochemical Properties
D-erythro-Imidazoleglycerol Phosphate Monohydrate is a crucial intermediate in the biosynthesis of histidine, an amino acid that plays a pivotal role in many biological processes . The imidazole ring, a notable component of this molecule, is key to the chemical’s participation in biochemical reactions involving nitrogen donors or acceptors .
Cellular Effects
The presence of the glycerol component in D-erythro-Imidazoleglycerol Phosphate Monohydrate allows for structural flexibility and solubility in biological environments, enhancing its utility in metabolic pathways . It is involved in the synthesis of nucleotides in cells and is a key intermediate in the dehydroketo acid synthesis pathway .
Molecular Mechanism
D-erythro-Imidazoleglycerol Phosphate Monohydrate can be synthesized from D-ribose 5-phosphate and formamidine . It is also involved in histidine biosynthesis .
Temporal Effects in Laboratory Settings
It is known that such intermediates are synthesized, manipulated, and degraded within cells .
Metabolic Pathways
D-erythro-Imidazoleglycerol Phosphate Monohydrate is involved in the biosynthesis of histidine . The study of this compound aids in understanding the regulation and function of the histidine biosynthesis pathway, providing insights into genetic regulation, enzyme mechanics, and potential points of metabolic control .
Méthodes De Préparation
Synthetic Routes and Reaction Conditions
The synthesis of D-erythro-Imidazoleglycerol Phosphate Monohydrate typically involves the reaction of D-ribose 5-phosphate with formamidine. The reaction conditions often require a controlled environment with specific temperature and pH levels to ensure the proper formation of the compound.
Industrial Production Methods
Industrial production methods for D-erythro-Imidazoleglycerol Phosphate Monohydrate are not extensively documented. the compound is generally produced in laboratory settings for research purposes. The production process involves the use of high-purity reagents and controlled reaction conditions to achieve the desired purity and yield .
Analyse Des Réactions Chimiques
Types of Reactions
D-erythro-Imidazoleglycerol Phosphate Monohydrate undergoes various chemical reactions, including:
Oxidation: The compound can be oxidized to form different products, depending on the reagents and conditions used.
Reduction: Reduction reactions can convert the compound into other derivatives.
Substitution: The imidazole ring and phosphate group can participate in substitution reactions with various reagents.
Common Reagents and Conditions
Common reagents used in the reactions of D-erythro-Imidazoleglycerol Phosphate Monohydrate include oxidizing agents like hydrogen peroxide, reducing agents like sodium borohydride, and various nucleophiles for substitution reactions .
Major Products Formed
The major products formed from the reactions of D-erythro-Imidazoleglycerol Phosphate Monohydrate depend on the specific reaction conditions. For example, oxidation can yield different phosphorylated imidazole derivatives, while reduction can produce glycerol derivatives .
Comparaison Avec Des Composés Similaires
Similar Compounds
Imidazoleglycerol Phosphate: Shares a similar structure but lacks the erythro configuration.
Histidinol Phosphate: Another intermediate in the histidine biosynthesis pathway.
Phosphoribosylformimino-5-aminoimidazole carboxamide ribotide (ProFAR): Involved in the purine biosynthesis pathway.
Uniqueness
D-erythro-Imidazoleglycerol Phosphate Monohydrate is unique due to its specific role in the histidine biosynthesis pathway and its distinct molecular structure. The erythro configuration and the presence of both the imidazole ring and phosphate group make it a valuable compound for biochemical research .
Activité Biologique
D-erythro-Imidazoleglycerol Phosphate Monohydrate (IGP) is a significant intermediate in the biosynthesis of histidine, an essential amino acid. This compound plays a crucial role in various biological processes across different organisms, including bacteria, yeast, and higher eukaryotes. This article delves into the biological activity of IGP, focusing on its metabolic pathways, enzymatic functions, and potential implications in health and disease.
1. Overview of D-erythro-Imidazoleglycerol Phosphate
D-erythro-Imidazoleglycerol Phosphate is a monoalkyl phosphate that exists in all living species. It is primarily involved in the histidine biosynthesis pathway , which consists of several enzymatic steps leading to the production of histidine from simpler precursors. In yeast (Saccharomyces cerevisiae), IGP is synthesized through the action of specific enzymes such as imidazole glycerol phosphate synthase (IGPS) and imidazoleglycerol-phosphate dehydratase (IGPD) .
2.1 Histidine Biosynthesis Pathway
The histidine biosynthesis pathway can be summarized as follows:
Key Enzymes:
- IGPS (HIS7 gene) : Catalyzes the first step; requires glutamine as a nitrogen source.
- IGPD (HIS3 gene) : Catalyzes the conversion of IGP to imidazole acetol phosphate, a crucial step in histidine metabolism .
3.1 Role in Metabolism
The activity of IGP is critical for maintaining adequate levels of histidine in organisms. Histidine is not only a building block for proteins but also serves as a precursor for several bioactive molecules, including histamine and carnosine. The regulation of IGP levels can impact various metabolic processes, including:
- Protein synthesis : Histidine availability affects overall protein synthesis rates.
- Cellular signaling : Histamine derived from histidine plays roles in immune response and neurotransmission.
3.2 Case Studies
A study investigating the metabolic profiles in Salmonella typhimurium mutants lacking IGPD revealed that these mutants exhibited impaired growth due to disruptions in histidine biosynthesis . This indicates that IGP and its associated enzymes are vital for bacterial survival and proliferation.
Another research highlighted the differential metabolite profiles during infections caused by Babesia microti, where alterations in histidine metabolism were observed, suggesting that IGP may play a role in host-pathogen interactions .
4. Inhibition and Therapeutic Potential
Certain inhibitors targeting IGPD have been explored for their potential use as herbicides since animals do not possess this enzyme. For instance, 3-Amino-1,2,4-triazole has been identified as a competitive inhibitor of IGPD, which may help control plant pathogens by disrupting their histidine biosynthesis .
5. Conclusion
D-erythro-Imidazoleglycerol Phosphate Monohydrate is an essential compound involved in the biosynthesis of histidine across various biological systems. Its enzymatic pathways are critical for maintaining metabolic homeostasis and influencing physiological processes. Further research into its biological activity could unveil new therapeutic targets for diseases related to amino acid metabolism.
Propriétés
IUPAC Name |
[2,3-dihydroxy-3-(4H-imidazol-4-yl)propyl] dihydrogen phosphate | |
---|---|---|
Source | PubChem | |
URL | https://pubchem.ncbi.nlm.nih.gov | |
Description | Data deposited in or computed by PubChem | |
InChI |
InChI=1S/C6H11N2O6P/c9-5(2-14-15(11,12)13)6(10)4-1-7-3-8-4/h1,3-6,9-10H,2H2,(H2,11,12,13) | |
Source | PubChem | |
URL | https://pubchem.ncbi.nlm.nih.gov | |
Description | Data deposited in or computed by PubChem | |
InChI Key |
SIVIZMCKQNFBMT-UHFFFAOYSA-N | |
Source | PubChem | |
URL | https://pubchem.ncbi.nlm.nih.gov | |
Description | Data deposited in or computed by PubChem | |
Canonical SMILES |
C1=NC=NC1C(C(COP(=O)(O)O)O)O | |
Source | PubChem | |
URL | https://pubchem.ncbi.nlm.nih.gov | |
Description | Data deposited in or computed by PubChem | |
Molecular Formula |
C6H11N2O6P | |
Source | PubChem | |
URL | https://pubchem.ncbi.nlm.nih.gov | |
Description | Data deposited in or computed by PubChem | |
DSSTOX Substance ID |
DTXSID90399950 | |
Record name | D-erythro-Imidazoleglycerol Phosphate Monohydrate | |
Source | EPA DSSTox | |
URL | https://comptox.epa.gov/dashboard/DTXSID90399950 | |
Description | DSSTox provides a high quality public chemistry resource for supporting improved predictive toxicology. | |
Molecular Weight |
238.14 g/mol | |
Source | PubChem | |
URL | https://pubchem.ncbi.nlm.nih.gov | |
Description | Data deposited in or computed by PubChem | |
CAS No. |
210241-69-3 | |
Record name | D-erythro-Imidazoleglycerol Phosphate Monohydrate | |
Source | EPA DSSTox | |
URL | https://comptox.epa.gov/dashboard/DTXSID90399950 | |
Description | DSSTox provides a high quality public chemistry resource for supporting improved predictive toxicology. | |
Avertissement et informations sur les produits de recherche in vitro
Veuillez noter que tous les articles et informations sur les produits présentés sur BenchChem sont destinés uniquement à des fins informatives. Les produits disponibles à l'achat sur BenchChem sont spécifiquement conçus pour des études in vitro, qui sont réalisées en dehors des organismes vivants. Les études in vitro, dérivées du terme latin "in verre", impliquent des expériences réalisées dans des environnements de laboratoire contrôlés à l'aide de cellules ou de tissus. Il est important de noter que ces produits ne sont pas classés comme médicaments et n'ont pas reçu l'approbation de la FDA pour la prévention, le traitement ou la guérison de toute condition médicale, affection ou maladie. Nous devons souligner que toute forme d'introduction corporelle de ces produits chez les humains ou les animaux est strictement interdite par la loi. Il est essentiel de respecter ces directives pour assurer la conformité aux normes légales et éthiques en matière de recherche et d'expérimentation.