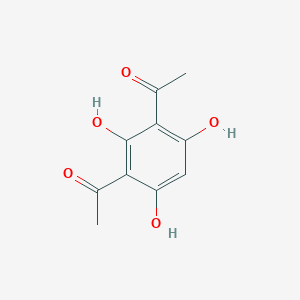
2,4-Diacetylphloroglucinol
Vue d'ensemble
Description
2,4-Diacetylphloroglucinol is a secondary metabolite produced by certain strains of Pseudomonas fluorescens. It is known for its broad-spectrum antibiotic activity against bacteria, fungi, oomycetes, and nematodes . This compound plays a significant role in the biocontrol of plant pathogens, making it a valuable asset in sustainable agriculture .
Applications De Recherche Scientifique
2,4-Diacetylphloroglucinol has a wide range of applications in scientific research:
Mécanisme D'action
Target of Action
2,4-Diacetylphloroglucinol (2,4-DAPG) is a secondary metabolite produced by certain strains of the Gram-negative bacterium Pseudomonas fluorescens . This compound is known for its broad-spectrum activity against various organisms, including plants, fungi, viruses, bacteria, and nematodes .
Mode of Action
The mode of action of 2,4-DAPG involves a rapid increase in membrane permeability . This action results in a reduction in the viability of the target organism, particularly in nutrient-poor environments .
Biochemical Pathways
The biosynthesis of 2,4-DAPG is regulated by a gene cluster termed phlACBDEFGH . The enzymes directly involved in the formation of 2,4-DAPG are encoded by the genes phlACBD located in a single operon . The gene phlD encodes a type III polyketide synthase which is responsible for the conversion of three molecules of malonyl-CoA to phloroglucinol (PG) . Then the acetyltransferase encoded by phlACB catalyzes the acetylation of PG to monoacetylphloroglucinol (MAPG) and 2,4-DAPG .
Pharmacokinetics
It is known that the phle gene is involved in the efflux of 2,4-dapg, and phle mutants are more sensitive to high concentrations of 2,4-dapg .
Result of Action
The result of 2,4-DAPG’s action is the inhibition of growth and reduction in the viability of the target organism . This is achieved through the disruption of the bacterial barrier structures . In addition, 2,4-DAPG has been shown to have antiviral, antimicrobial, anti-peronosporomycetes, ichthyotoxic, insect and mammal anti-feedant, anti-helminthic, phytotoxic, antioxidant, cytotoxic, antitumor and plant-growth-regulating activities .
Action Environment
The action of 2,4-DAPG can be influenced by environmental factors. For instance, the antibiotic’s action involves a rapid increase in membrane permeability and a reduction in its viability in nutrient-poor environments . Furthermore, divalent cations such as Zn 2+, Cu 2+ and NH 4 Mo 2+ have been found to stimulate the production of DAPG .
Analyse Biochimique
Biochemical Properties
2,4-Diacetylphloroglucinol interacts with various enzymes and proteins. The key synthases coded by the phlACBD gene cluster from the strain Pseudomonas fluorescens CHA0 are involved in its production . The gene phlD encodes a type III polyketide synthase responsible for the conversion of three molecules of malonyl-CoA to phloroglucinol .
Cellular Effects
This compound has been shown to have significant effects on various types of cells and cellular processes. For instance, it causes different stages of disorganization in hyphal tips of Pythium ultimum var. sporangiiferum, including alteration of the plasma membrane, vacuolization, and cell content disintegration .
Molecular Mechanism
The molecular mechanism of this compound involves binding interactions with biomolecules, enzyme inhibition or activation, and changes in gene expression . For example, it has been found that this compound reduces Beta-Amyloid production and secretion by regulating ADAM10 and intracellular trafficking .
Temporal Effects in Laboratory Settings
Over time, the effects of this compound can change in laboratory settings. For instance, the concentration of this compound was found to be 179 mg/L after induction for 36 h by fed-batch fermentation .
Dosage Effects in Animal Models
In animal models, the effects of this compound can vary with different dosages
Metabolic Pathways
This compound is involved in several metabolic pathways. The biosynthesis of 2,4-DAPG is regulated by a gene cluster termed phlACBDEFGH . The enzymes directly involved in the formation of 2,4-DAPG are encoded by the genes phlACBD located in a single operon .
Méthodes De Préparation
Synthetic Routes and Reaction Conditions: The synthesis of 2,4-Diacetylphloroglucinol can be achieved through the Friedel-Crafts acylation of phloroglucinol using a silica sulphuric acid catalyst. This method involves ultrasound-assisted synthesis under solvent-free conditions, yielding a high purity product . The reaction is typically carried out at 60°C for 15-20 minutes with a 10% (w/w) catalyst .
Industrial Production Methods: In an industrial setting, this compound can be produced using engineered Escherichia coli. The key synthases encoded by the phlACBD gene cluster from Pseudomonas fluorescens are overexpressed in E. coli, along with additional genes to enhance biosynthesis . Optimized fermentation conditions in a bioreactor can yield significant concentrations of the compound .
Analyse Des Réactions Chimiques
Types of Reactions: 2,4-Diacetylphloroglucinol undergoes various chemical reactions, including oxidation, reduction, and substitution.
Common Reagents and Conditions:
Oxidation: This reaction can be facilitated by oxidizing agents such as hydrogen peroxide or potassium permanganate.
Reduction: Reducing agents like sodium borohydride can be used to reduce this compound.
Substitution: Substitution reactions often involve nucleophiles such as amines or alcohols.
Major Products: The major products formed from these reactions depend on the specific reagents and conditions used. For example, oxidation may yield quinones, while reduction can produce alcohol derivatives.
Comparaison Avec Des Composés Similaires
- Pyoluteorin
- Pyrrolnitrin
- Phenazine
Comparison: While all these compounds exhibit antimicrobial properties, 2,4-Diacetylphloroglucinol is unique due to its broad-spectrum activity and its ability to be produced by engineered microorganisms . Additionally, its diverse applications in agriculture, medicine, and biotechnology set it apart from other similar compounds .
Activité Biologique
2,4-Diacetylphloroglucinol (2,4-DAPG) is a secondary metabolite produced by certain strains of Pseudomonas fluorescens, known for its significant biological activities, particularly in plant protection and antimicrobial properties. This compound has garnered attention due to its ability to inhibit a wide range of plant pathogens, including fungi, bacteria, and nematodes. This article explores the biological activity of 2,4-DAPG, focusing on its mechanisms of action, effects on various organisms, and potential applications in agriculture and medicine.
The biological activity of 2,4-DAPG is primarily attributed to its antimicrobial properties. Research has demonstrated that 2,4-DAPG exerts its effects through several mechanisms:
- Disruption of Membrane Integrity : 2,4-DAPG increases the permeability of bacterial membranes, leading to cell lysis and death. Studies using atomic force microscopy revealed that exposure to 2,4-DAPG caused significant disturbances in the outer membrane of Escherichia coli and Staphylococcus aureus, resulting in intracellular leakage and loss of viability over time .
- Inhibition of Biofilm Formation : 2,4-DAPG has shown potential in inhibiting biofilm formation in various bacterial species. Its antibiofilm activity is concentration-dependent, with higher concentrations leading to more significant reductions in biofilm metabolic activity .
- Quorum Sensing Inhibition : At subinhibitory concentrations, 2,4-DAPG can interfere with quorum sensing mechanisms in bacteria such as Pectobacterium carotovorum, reducing the biosynthesis of signaling molecules like acyl-homoserine lactones .
- Proton Ionophore Activity : In yeast models like Saccharomyces cerevisiae, 2,4-DAPG acts as a proton ionophore that dissipates the proton gradient across mitochondrial membranes. This uncoupling leads to growth inhibition by disrupting ATP synthesis .
Antimicrobial Activity
The antimicrobial spectrum of 2,4-DAPG is broad:
Pathogen Type | Pathogen Examples | Activity Type |
---|---|---|
Bacteria | E. coli, S. aureus | Bactericidal |
Fungi | Gaeumannomyces graminis, Verticillium dahliae | Fungicidal |
Oomycetes | Pythium ultimum | Growth Inhibition |
Nematodes | Various species | Nematicidal |
Studies have shown that strains of Pseudomonas fluorescens producing 2,4-DAPG can effectively suppress these pathogens through direct antimicrobial action .
Case Studies
- Inhibition of Plant Pathogens : A study demonstrated that 2,4-DAPG significantly inhibited the growth of various phytopathogenic fungi. For instance, it reduced the growth rates of Gaeumannomyces graminis by up to 70% at certain concentrations .
- Biofilm Disruption : Another research highlighted that treatment with 2,4-DAPG led to a marked decrease in biofilm formation by S. aureus. The metabolic activity within biofilms was reduced by over 50% when treated with sub-MIC concentrations over a 24-hour period .
- Effect on Quorum Sensing : In experiments with Pectobacterium carotovorum, subinhibitory levels of 2,4-DAPG were found to significantly lower the production of acyl-homoserine lactones, indicating its potential use as a biocontrol agent against soft rot diseases in crops .
Structural Modifications and Analogues
Recent studies have explored the synthesis of analogues of 2,4-DAPG to enhance its biological activity. For example:
- Novel Analogues : Fourteen new analogues were synthesized and tested against common fungal pathogens like Penicillium digitatum. Some analogues exhibited antifungal activities significantly higher than those of 2,4-DAPG itself .
Analogue ID | Antifungal Activity (%) against P. digitatum at 25 µg/mL |
---|---|
MP1 | 29.7 |
MP3 | 80.0 |
MP4 | 84.0 |
These modifications aim to optimize the amphiphilic properties and improve efficacy against target organisms while minimizing potential side effects.
Propriétés
IUPAC Name |
1-(3-acetyl-2,4,6-trihydroxyphenyl)ethanone | |
---|---|---|
Source | PubChem | |
URL | https://pubchem.ncbi.nlm.nih.gov | |
Description | Data deposited in or computed by PubChem | |
InChI |
InChI=1S/C10H10O5/c1-4(11)8-6(13)3-7(14)9(5(2)12)10(8)15/h3,13-15H,1-2H3 | |
Source | PubChem | |
URL | https://pubchem.ncbi.nlm.nih.gov | |
Description | Data deposited in or computed by PubChem | |
InChI Key |
PIFFQYJYNWXNGE-UHFFFAOYSA-N | |
Source | PubChem | |
URL | https://pubchem.ncbi.nlm.nih.gov | |
Description | Data deposited in or computed by PubChem | |
Canonical SMILES |
CC(=O)C1=C(C(=C(C=C1O)O)C(=O)C)O | |
Source | PubChem | |
URL | https://pubchem.ncbi.nlm.nih.gov | |
Description | Data deposited in or computed by PubChem | |
Molecular Formula |
C10H10O5 | |
Source | PubChem | |
URL | https://pubchem.ncbi.nlm.nih.gov | |
Description | Data deposited in or computed by PubChem | |
DSSTOX Substance ID |
DTXSID10175995 | |
Record name | 2,4-Diacetylphloroglucinol | |
Source | EPA DSSTox | |
URL | https://comptox.epa.gov/dashboard/DTXSID10175995 | |
Description | DSSTox provides a high quality public chemistry resource for supporting improved predictive toxicology. | |
Molecular Weight |
210.18 g/mol | |
Source | PubChem | |
URL | https://pubchem.ncbi.nlm.nih.gov | |
Description | Data deposited in or computed by PubChem | |
CAS No. |
2161-86-6 | |
Record name | 2,4-Diacetylphloroglucinol | |
Source | CAS Common Chemistry | |
URL | https://commonchemistry.cas.org/detail?cas_rn=2161-86-6 | |
Description | CAS Common Chemistry is an open community resource for accessing chemical information. Nearly 500,000 chemical substances from CAS REGISTRY cover areas of community interest, including common and frequently regulated chemicals, and those relevant to high school and undergraduate chemistry classes. This chemical information, curated by our expert scientists, is provided in alignment with our mission as a division of the American Chemical Society. | |
Explanation | The data from CAS Common Chemistry is provided under a CC-BY-NC 4.0 license, unless otherwise stated. | |
Record name | 2,4-Diacetylphloroglucinol | |
Source | ChemIDplus | |
URL | https://pubchem.ncbi.nlm.nih.gov/substance/?source=chemidplus&sourceid=0002161866 | |
Description | ChemIDplus is a free, web search system that provides access to the structure and nomenclature authority files used for the identification of chemical substances cited in National Library of Medicine (NLM) databases, including the TOXNET system. | |
Record name | 2,4-Diacetylphloroglucinol | |
Source | EPA DSSTox | |
URL | https://comptox.epa.gov/dashboard/DTXSID10175995 | |
Description | DSSTox provides a high quality public chemistry resource for supporting improved predictive toxicology. | |
Record name | 2,4-Diacetylbenzene-1,3,5-triol | |
Source | European Chemicals Agency (ECHA) | |
URL | https://echa.europa.eu/information-on-chemicals | |
Description | The European Chemicals Agency (ECHA) is an agency of the European Union which is the driving force among regulatory authorities in implementing the EU's groundbreaking chemicals legislation for the benefit of human health and the environment as well as for innovation and competitiveness. | |
Explanation | Use of the information, documents and data from the ECHA website is subject to the terms and conditions of this Legal Notice, and subject to other binding limitations provided for under applicable law, the information, documents and data made available on the ECHA website may be reproduced, distributed and/or used, totally or in part, for non-commercial purposes provided that ECHA is acknowledged as the source: "Source: European Chemicals Agency, http://echa.europa.eu/". Such acknowledgement must be included in each copy of the material. ECHA permits and encourages organisations and individuals to create links to the ECHA website under the following cumulative conditions: Links can only be made to webpages that provide a link to the Legal Notice page. | |
Record name | 2,4-DIACETYLPHLOROGLUCINOL | |
Source | FDA Global Substance Registration System (GSRS) | |
URL | https://gsrs.ncats.nih.gov/ginas/app/beta/substances/8XV4YYO3WN | |
Description | The FDA Global Substance Registration System (GSRS) enables the efficient and accurate exchange of information on what substances are in regulated products. Instead of relying on names, which vary across regulatory domains, countries, and regions, the GSRS knowledge base makes it possible for substances to be defined by standardized, scientific descriptions. | |
Explanation | Unless otherwise noted, the contents of the FDA website (www.fda.gov), both text and graphics, are not copyrighted. They are in the public domain and may be republished, reprinted and otherwise used freely by anyone without the need to obtain permission from FDA. Credit to the U.S. Food and Drug Administration as the source is appreciated but not required. | |
Retrosynthesis Analysis
AI-Powered Synthesis Planning: Our tool employs the Template_relevance Pistachio, Template_relevance Bkms_metabolic, Template_relevance Pistachio_ringbreaker, Template_relevance Reaxys, Template_relevance Reaxys_biocatalysis model, leveraging a vast database of chemical reactions to predict feasible synthetic routes.
One-Step Synthesis Focus: Specifically designed for one-step synthesis, it provides concise and direct routes for your target compounds, streamlining the synthesis process.
Accurate Predictions: Utilizing the extensive PISTACHIO, BKMS_METABOLIC, PISTACHIO_RINGBREAKER, REAXYS, REAXYS_BIOCATALYSIS database, our tool offers high-accuracy predictions, reflecting the latest in chemical research and data.
Strategy Settings
Precursor scoring | Relevance Heuristic |
---|---|
Min. plausibility | 0.01 |
Model | Template_relevance |
Template Set | Pistachio/Bkms_metabolic/Pistachio_ringbreaker/Reaxys/Reaxys_biocatalysis |
Top-N result to add to graph | 6 |
Feasible Synthetic Routes
A: While the precise target of 2,4-DAPG is not fully understood, research suggests that its bactericidal action stems from the disruption of bacterial barrier structures like the cell membrane []. This can lead to increased membrane permeability, ultimately resulting in cell death, particularly in nutrient-poor environments []. In fungal pathogens like Pythium ultimum, exposure to 2,4-DAPG causes disrupted mycelium formation and a significant increase in glutathione, a protective tripeptide, indicating cellular stress response to the compound [].
A: 2,4-DAPG production by Pseudomonas fluorescens is influenced by environmental factors. For instance, chloride ions stimulate the production of the antibiotic pyoluteorin, which in turn, represses 2,4-DAPG biosynthesis []. Additionally, the nitrogen source in growth media significantly affects 2,4-DAPG production, with organic nitrogen being essential []. These findings highlight the importance of environmental conditions for the production and potential efficacy of 2,4-DAPG.
ANone: 2,4-DAPG itself is not typically used as a catalyst. Its primary role is as an antimicrobial agent. It exhibits broad-spectrum activity against various plant pathogens, making it a valuable tool for biocontrol.
A: Yes, computational docking studies have been performed in conjunction with the crystal structure analysis of the enzyme PhlG, which degrades 2,4-DAPG. These studies helped elucidate the active site and zinc-dependent hydrolysis mechanism of PhlG [].
A: The stability of 2,4-DAPG can be influenced by various factors. It appears to be degraded to monoacetylphloroglucinol by the bacterium Pseudomonas fluorescens CHA0 itself, potentially impacting its long-term efficacy []. Formulations incorporating mannitol as a carbon source in a bean-based matrix have shown promise in enhancing the biocontrol efficacy of a 2,4-DAPG-producing Pseudomonas strain against tomato Fusarium wilt []. This approach highlights the potential for optimizing formulations to improve the delivery and effectiveness of 2,4-DAPG-producing biocontrol agents.
ANone: Specific SHE regulations for 2,4-DAPG are not discussed in the provided research. As a naturally occurring compound, its use as a biocontrol agent would likely fall under regulations governing biopesticides.
ANone: The provided research primarily focuses on the role of 2,4-DAPG in biocontrol within the rhizosphere, with limited information on its detailed pharmacokinetics in plants or other organisms.
A: Numerous studies demonstrate the in vitro and in vivo efficacy of 2,4-DAPG against various plant pathogens. For instance, in vitro assays show its inhibitory effects on Thielaviopsis basicola, the causal agent of black root rot in tobacco, and Gaeumannomyces graminis var. tritici, responsible for take-all disease in wheat []. Greenhouse experiments further support its role in suppressing these diseases in tobacco and wheat [].
A: Fusarium oxysporum, a fungal pathogen, displays varying levels of sensitivity to 2,4-DAPG, with some strains exhibiting tolerance. Biochemical analysis revealed that a subset of these tolerant strains could metabolize 2,4-DAPG, suggesting a potential resistance mechanism [].
ANone: The research primarily focuses on the biocontrol applications of 2,4-DAPG. While it demonstrates efficacy against plant pathogens, comprehensive toxicological data on its effects on humans or other organisms are not provided in these studies.
A: The research highlights the importance of optimizing formulations to enhance the biocontrol efficacy of 2,4-DAPG-producing bacteria. For instance, amending a bean-based formulation with mannitol as a carbon source improved its performance against tomato Fusarium wilt []. This suggests that formulation strategies can influence the delivery and effectiveness of 2,4-DAPG in the rhizosphere.
A: The research highlights the significant contribution of 2,4-DAPG-producing Pseudomonas fluorescens strains in the natural suppression of soilborne plant diseases. Early studies identified 2,4-DAPG as a key factor in the biocontrol activity of these bacteria [], and subsequent research has explored its biosynthesis, regulation, and efficacy against various plant pathogens. The identification of genes involved in 2,4-DAPG biosynthesis [, ], the characterization of regulatory mechanisms [, ], and the development of methods to quantify 2,4-DAPG producers in the rhizosphere [, ] represent significant milestones in the field.
A: Research on 2,4-DAPG and its producing bacteria involves collaborations across multiple disciplines, including microbiology, plant pathology, genetics, biochemistry, and ecology. This collaborative approach is evident in the diverse research areas covered, ranging from the molecular mechanisms of 2,4-DAPG biosynthesis and regulation to its ecological role in disease suppressive soils and the development of biocontrol applications [, , , , , , , , , ]. This interdisciplinary approach is crucial for advancing our understanding of this important biocontrol agent and its potential for sustainable agriculture.
Avertissement et informations sur les produits de recherche in vitro
Veuillez noter que tous les articles et informations sur les produits présentés sur BenchChem sont destinés uniquement à des fins informatives. Les produits disponibles à l'achat sur BenchChem sont spécifiquement conçus pour des études in vitro, qui sont réalisées en dehors des organismes vivants. Les études in vitro, dérivées du terme latin "in verre", impliquent des expériences réalisées dans des environnements de laboratoire contrôlés à l'aide de cellules ou de tissus. Il est important de noter que ces produits ne sont pas classés comme médicaments et n'ont pas reçu l'approbation de la FDA pour la prévention, le traitement ou la guérison de toute condition médicale, affection ou maladie. Nous devons souligner que toute forme d'introduction corporelle de ces produits chez les humains ou les animaux est strictement interdite par la loi. Il est essentiel de respecter ces directives pour assurer la conformité aux normes légales et éthiques en matière de recherche et d'expérimentation.