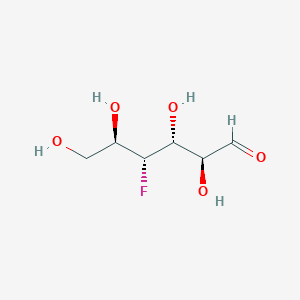
4-Deoxy-4-fluoro-D-mannose
Vue d'ensemble
Description
4-Deoxy-4-fluoro-D-mannose is a fundamental constituent in biomedicine and plays a significant role in the advancement of antiviral pharmaceuticals . Its exceptional molecular composition allows it to precisely pinpoint and impede the duplication of specific viruses, such as the influenza virus .
Synthesis Analysis
4-Deoxy-4-fluoro-D-mannose is synthesized by the transfer of a 6-hydroxyl group from 6,6’-dideoxyadenosine to the C6 hydroxyl group of 3,6’-dihexadecylthio adenosine .Molecular Structure Analysis
The molecular formula of 4-Deoxy-4-fluoro-D-mannose is C6H11FO5 . It has an average mass of 182.147 Da and a monoisotopic mass of 182.059052 Da .Chemical Reactions Analysis
4-Deoxy-4-fluoro-D-mannose inhibits the glycosylation of the G protein of vesicular stomatitis virus . It blocks the synthesis of lipid-linked oligosaccharides .Physical And Chemical Properties Analysis
The physical and chemical properties of 4-Deoxy-4-fluoro-D-mannose are influenced by its high electronegativity and the absence of any other substituent . The C–F bond is highly polarized, which affects the electron density of the carbohydrate structure .Applications De Recherche Scientifique
Inhibition of Glycosylation of Viral G-Protein
4-Deoxy-4-fluoro-D-mannose (4F-Man) has been shown to inhibit the glycosylation of the G protein of vesicular stomatitis virus . This inhibition is a consequence of depleted intracellular pools of Glc3Man9GlcNAc2-PP-Dol .
Interference with Viral Duplication
4F-Man’s unique molecular composition allows it to precisely pinpoint and impede the duplication of specific viruses, such as the influenza virus .
Metabolization in Yeast
4F-Man has been shown to be metabolized in yeast . This could potentially open up new avenues for research in yeast metabolism and bioengineering.
Molecular Recognition Studies
Fluorinated carbohydrates like 4F-Man have been used as chemical probes for molecular recognition studies . These studies can provide valuable insights into the interactions between molecules.
Development of Antiviral Pharmaceuticals
Due to its ability to interfere with viral duplication, 4F-Man plays a crucial role in the development of antiviral pharmaceuticals .
Study of Protein Glycosylation
4F-Man has been used to study the biosynthesis and function of glycoprotein oligosaccharide chains . This research could potentially lead to the development of new therapeutic strategies for diseases related to protein glycosylation.
Mécanisme D'action
Target of Action
The primary target of 4-Deoxy-4-fluoro-D-mannose (4F-Man) is the glycoprotein (G) of vesicular stomatitis virus . This glycoprotein plays a crucial role in the life cycle of the virus, particularly in the process of glycosylation .
Mode of Action
4F-Man, a synthetic analog of D-mannose, interacts with its target by inhibiting the glycosylation of the G protein . When cells infected with vesicular stomatitis virus are exposed to 4F-Man, the G protein migrates more rapidly than G protein isolated from control cells . This indicates that 4F-Man blocks the glycosylation of the G protein .
Biochemical Pathways
The effect of 4F-Man on the synthesis of the G protein affects the biosynthesis and function of the asparagine-linked oligosaccharide chains of glycoproteins . This inhibition is a consequence of depleted intracellular pools of Glc3Man9GlcNAc2-PP-Dol . Additionally, incubation of Saccharomyces cerevisiae S288C with 4F-Man resulted in the formation of three metabolites: 4-deoxy-4-fluoro-D-mannose 1,6-bisphosphate, 4-deoxy-4-fluoro-D-mannose 6-phosphate, and GDP-4-deoxy-4-fluoro-D-mannose .
Pharmacokinetics
The pharmacokinetics of 4F-Man involve its interaction with the cells infected with vesicular stomatitis virus. The cells are incubated with 4F-Man for a certain period, after which the viral proteins are analyzed . .
Result of Action
The molecular effect of 4F-Man’s action is the inhibition of the glycosylation of the G protein, which alters the normal events of protein glycosylation . On a cellular level, this results in the rapid migration of the G protein from cells exposed to 4F-Man .
Action Environment
The action of 4F-Man is influenced by the environment within the cells infected with vesicular stomatitis virus. The concentration of 4F-Man, the duration of incubation, and the presence of other compounds like tunicamycin can affect the efficacy of 4F-Man . .
Safety and Hazards
Orientations Futures
Propriétés
IUPAC Name |
(2S,3R,4R,5R)-4-fluoro-2,3,5,6-tetrahydroxyhexanal | |
---|---|---|
Source | PubChem | |
URL | https://pubchem.ncbi.nlm.nih.gov | |
Description | Data deposited in or computed by PubChem | |
InChI |
InChI=1S/C6H11FO5/c7-5(3(10)1-8)6(12)4(11)2-9/h2-6,8,10-12H,1H2/t3-,4-,5-,6-/m1/s1 | |
Source | PubChem | |
URL | https://pubchem.ncbi.nlm.nih.gov | |
Description | Data deposited in or computed by PubChem | |
InChI Key |
GCEGLMFBNPWYQO-KVTDHHQDSA-N | |
Source | PubChem | |
URL | https://pubchem.ncbi.nlm.nih.gov | |
Description | Data deposited in or computed by PubChem | |
Canonical SMILES |
C(C(C(C(C(C=O)O)O)F)O)O | |
Source | PubChem | |
URL | https://pubchem.ncbi.nlm.nih.gov | |
Description | Data deposited in or computed by PubChem | |
Isomeric SMILES |
C([C@H]([C@H]([C@@H]([C@@H](C=O)O)O)F)O)O | |
Source | PubChem | |
URL | https://pubchem.ncbi.nlm.nih.gov | |
Description | Data deposited in or computed by PubChem | |
Molecular Formula |
C6H11FO5 | |
Source | PubChem | |
URL | https://pubchem.ncbi.nlm.nih.gov | |
Description | Data deposited in or computed by PubChem | |
DSSTOX Substance ID |
DTXSID70236617 | |
Record name | 4-Deoxy-4-fluoromannose | |
Source | EPA DSSTox | |
URL | https://comptox.epa.gov/dashboard/DTXSID70236617 | |
Description | DSSTox provides a high quality public chemistry resource for supporting improved predictive toxicology. | |
Molecular Weight |
182.15 g/mol | |
Source | PubChem | |
URL | https://pubchem.ncbi.nlm.nih.gov | |
Description | Data deposited in or computed by PubChem | |
Product Name |
4-Deoxy-4-fluoro-D-mannose | |
CAS RN |
87764-47-4 | |
Record name | 4-Deoxy-4-fluoromannose | |
Source | ChemIDplus | |
URL | https://pubchem.ncbi.nlm.nih.gov/substance/?source=chemidplus&sourceid=0087764474 | |
Description | ChemIDplus is a free, web search system that provides access to the structure and nomenclature authority files used for the identification of chemical substances cited in National Library of Medicine (NLM) databases, including the TOXNET system. | |
Record name | 4-Deoxy-4-fluoromannose | |
Source | EPA DSSTox | |
URL | https://comptox.epa.gov/dashboard/DTXSID70236617 | |
Description | DSSTox provides a high quality public chemistry resource for supporting improved predictive toxicology. | |
Retrosynthesis Analysis
AI-Powered Synthesis Planning: Our tool employs the Template_relevance Pistachio, Template_relevance Bkms_metabolic, Template_relevance Pistachio_ringbreaker, Template_relevance Reaxys, Template_relevance Reaxys_biocatalysis model, leveraging a vast database of chemical reactions to predict feasible synthetic routes.
One-Step Synthesis Focus: Specifically designed for one-step synthesis, it provides concise and direct routes for your target compounds, streamlining the synthesis process.
Accurate Predictions: Utilizing the extensive PISTACHIO, BKMS_METABOLIC, PISTACHIO_RINGBREAKER, REAXYS, REAXYS_BIOCATALYSIS database, our tool offers high-accuracy predictions, reflecting the latest in chemical research and data.
Strategy Settings
Precursor scoring | Relevance Heuristic |
---|---|
Min. plausibility | 0.01 |
Model | Template_relevance |
Template Set | Pistachio/Bkms_metabolic/Pistachio_ringbreaker/Reaxys/Reaxys_biocatalysis |
Top-N result to add to graph | 6 |
Feasible Synthetic Routes
Q & A
Q1: How does 4-Deoxy-4-fluoro-D-mannose disrupt protein glycosylation?
A1: 4FMan exerts its effect by interfering with the dolichol pathway, a critical stage in N-linked glycosylation. Specifically, its guanosine diphosphate ester (GDP-4FMan) competitively inhibits the enzyme responsible for adding mannose units to the growing oligosaccharide chain on dolichol pyrophosphate. [] This blockage primarily occurs during the formation of Dol-PP-(GlcNAc)2Man2, leading to truncated lipid-linked oligosaccharides. [] While 4FMan is metabolized into GDP-4FMan and Dol-P-4FMan within cells, both exhibit limited substrate activity with relevant enzymes, further contributing to the disruption of normal glycosylation. []
Q2: How does the action of 4-Deoxy-4-fluoro-D-mannose differ from 3-Deoxy-3-fluoro-D-mannose in Saccharomyces cerevisiae?
A2: While both 4FMan and 3-deoxy-3-fluoro-D-mannose (3FMan) are mannose analogues, they demonstrate differing levels of uptake and metabolic conversion in Saccharomyces cerevisiae. 4FMan is readily transported into yeast cells and efficiently converted into its respective sugar nucleotides, including 4-deoxy-4-fluoro-D-mannose 1,6-bisphosphate, 4-deoxy-4-fluoro-D-mannose 6-phosphate, and GDP-4FMan. [] This ultimately leads to the incorporation of radioactive material from 4FMan into cell wall polysaccharides. [] Conversely, 3FMan exhibits poorer transport into yeast cells and less efficient conversion into sugar nucleotides, resulting in significantly less incorporation into the cell wall fraction. []
Avertissement et informations sur les produits de recherche in vitro
Veuillez noter que tous les articles et informations sur les produits présentés sur BenchChem sont destinés uniquement à des fins informatives. Les produits disponibles à l'achat sur BenchChem sont spécifiquement conçus pour des études in vitro, qui sont réalisées en dehors des organismes vivants. Les études in vitro, dérivées du terme latin "in verre", impliquent des expériences réalisées dans des environnements de laboratoire contrôlés à l'aide de cellules ou de tissus. Il est important de noter que ces produits ne sont pas classés comme médicaments et n'ont pas reçu l'approbation de la FDA pour la prévention, le traitement ou la guérison de toute condition médicale, affection ou maladie. Nous devons souligner que toute forme d'introduction corporelle de ces produits chez les humains ou les animaux est strictement interdite par la loi. Il est essentiel de respecter ces directives pour assurer la conformité aux normes légales et éthiques en matière de recherche et d'expérimentation.