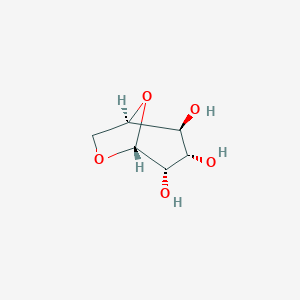
1,6-Anhydro-beta-D-mannopyranose
Vue d'ensemble
Description
1,6-Anhydro-beta-D-mannopyranose is a major organic tracer generated by burned cellulose . This compound is one of several that are used to evaluate, in atmospheric samples, the burning of wood .
Synthesis Analysis
1,6-Anhydro-beta-D-mannopyranose is a naturally occurring anhydro sugar with gustatory properties. It serves as an essential building block in the biosynthesis of glycoproteins and glycolipids found in both plant and animal tissues . Moreover, this monosaccharide has been utilized in drug synthesis and holds potential therapeutic applications for cancer, inflammation, and autoimmune diseases .Molecular Structure Analysis
The molecular formula of 1,6-Anhydro-beta-D-mannopyranose is C6H10O5 . Its molecular weight is 162.14 .Physical And Chemical Properties Analysis
The physical and chemical properties of 1,6-Anhydro-beta-D-mannopyranose include a molecular weight of 162.14 and a molecular formula of C6H10O5 .Applications De Recherche Scientifique
High-Pressure Dielectric Studies
1,6-Anhydro-beta-D-mannopyranose has been used in high-pressure dielectric studies . Broadband Dielectric Spectroscopy was applied to investigate the molecular dynamics of 1,6-anhydro-beta-D-mannopyranose (a hydrogen-bonded system) at different thermodynamic conditions . This research helps to understand the impact of compression on the fragility, Temperature-Pressure Superpositioning and pressure coefficient of the glassy crystal/glass transition temperatures .
Organic Tracer for Burned Cellulose
1,6-Anhydro-beta-D-mannopyranose is a major organic tracer generated by burned cellulose . This compound is one of several that are used to evaluate, in atmospheric samples, the burning of wood . This application is particularly useful in environmental science and atmospheric chemistry for tracking the source of particulate matter in the air.
Chemical Synthesis
1,6-Anhydro-beta-D-mannopyranose is also used in chemical synthesis . It is available from chemical suppliers and can be used as a starting material or intermediate in the synthesis of other chemicals .
Safety and Hazards
Mécanisme D'action
Target of Action
1,6-Anhydro-beta-D-mannopyranose is a major organic tracer generated by burned cellulose . This compound is one of several that are used to evaluate, in atmospheric samples, the burning of wood .
Mode of Action
It is postulated that this monosaccharide binds to specific cell receptors, triggering signaling cascades that result in the synthesis of proteins and other molecules .
Biochemical Pathways
Given its role as a major organic tracer in the burning of wood, it may be involved in the biochemical pathways related to cellulose combustion .
Result of Action
It is known that this compound is used to evaluate the burning of wood in atmospheric samples , suggesting that it may have a role in monitoring and assessing environmental changes related to combustion processes.
Action Environment
The action of 1,6-Anhydro-beta-D-mannopyranose is influenced by environmental factors, particularly those related to the burning of wood . The efficacy and stability of this compound may vary depending on the specific conditions of the environment, such as temperature, humidity, and the presence of other chemical compounds.
Propriétés
IUPAC Name |
(1R,2S,3S,4S,5R)-6,8-dioxabicyclo[3.2.1]octane-2,3,4-triol | |
---|---|---|
Source | PubChem | |
URL | https://pubchem.ncbi.nlm.nih.gov | |
Description | Data deposited in or computed by PubChem | |
InChI |
InChI=1S/C6H10O5/c7-3-2-1-10-6(11-2)5(9)4(3)8/h2-9H,1H2/t2-,3-,4+,5+,6-/m1/s1 | |
Source | PubChem | |
URL | https://pubchem.ncbi.nlm.nih.gov | |
Description | Data deposited in or computed by PubChem | |
InChI Key |
TWNIBLMWSKIRAT-RWOPYEJCSA-N | |
Source | PubChem | |
URL | https://pubchem.ncbi.nlm.nih.gov | |
Description | Data deposited in or computed by PubChem | |
Canonical SMILES |
C1C2C(C(C(C(O1)O2)O)O)O | |
Source | PubChem | |
URL | https://pubchem.ncbi.nlm.nih.gov | |
Description | Data deposited in or computed by PubChem | |
Isomeric SMILES |
C1[C@@H]2[C@H]([C@@H]([C@@H]([C@H](O1)O2)O)O)O | |
Source | PubChem | |
URL | https://pubchem.ncbi.nlm.nih.gov | |
Description | Data deposited in or computed by PubChem | |
Molecular Formula |
C6H10O5 | |
Source | PubChem | |
URL | https://pubchem.ncbi.nlm.nih.gov | |
Description | Data deposited in or computed by PubChem | |
DSSTOX Substance ID |
DTXSID101016516 | |
Record name | 1,6-Anhydro-D-mannose | |
Source | EPA DSSTox | |
URL | https://comptox.epa.gov/dashboard/DTXSID101016516 | |
Description | DSSTox provides a high quality public chemistry resource for supporting improved predictive toxicology. | |
Molecular Weight |
162.14 g/mol | |
Source | PubChem | |
URL | https://pubchem.ncbi.nlm.nih.gov | |
Description | Data deposited in or computed by PubChem | |
Product Name |
1,6-Anhydro-beta-D-mannopyranose | |
CAS RN |
14168-65-1 | |
Record name | 1,6-Anhydro-D-mannose | |
Source | EPA DSSTox | |
URL | https://comptox.epa.gov/dashboard/DTXSID101016516 | |
Description | DSSTox provides a high quality public chemistry resource for supporting improved predictive toxicology. | |
Retrosynthesis Analysis
AI-Powered Synthesis Planning: Our tool employs the Template_relevance Pistachio, Template_relevance Bkms_metabolic, Template_relevance Pistachio_ringbreaker, Template_relevance Reaxys, Template_relevance Reaxys_biocatalysis model, leveraging a vast database of chemical reactions to predict feasible synthetic routes.
One-Step Synthesis Focus: Specifically designed for one-step synthesis, it provides concise and direct routes for your target compounds, streamlining the synthesis process.
Accurate Predictions: Utilizing the extensive PISTACHIO, BKMS_METABOLIC, PISTACHIO_RINGBREAKER, REAXYS, REAXYS_BIOCATALYSIS database, our tool offers high-accuracy predictions, reflecting the latest in chemical research and data.
Strategy Settings
Precursor scoring | Relevance Heuristic |
---|---|
Min. plausibility | 0.01 |
Model | Template_relevance |
Template Set | Pistachio/Bkms_metabolic/Pistachio_ringbreaker/Reaxys/Reaxys_biocatalysis |
Top-N result to add to graph | 6 |
Feasible Synthetic Routes
Q & A
Q1: Can 1,6-Anhydro-beta-D-mannopyranose derivatives be used to synthesize complex molecules?
A1: Yes, 1,6-Anhydro-beta-D-mannopyranose derivatives have proven to be valuable building blocks in organic synthesis. For instance, ketoxime ethers at the C3 position of these derivatives can act as radical traps, enabling the synthesis of advanced intermediates for complex molecules like (-)-tetrodotoxin. [] This highlights the potential of these derivatives in constructing intricate molecular structures relevant to various fields, including medicinal chemistry.
Q2: Are there any known instances of 1,6-Anhydro-beta-D-mannopyranose derivatives exhibiting biological activity?
A2: Research suggests that certain modifications to the 1,6-Anhydro-beta-D-mannopyranose structure can lead to compounds with enzyme inhibitory activity. Specifically, methyl acarviosin analogues incorporating the 1,6-anhydro-beta-D-mannopyranose residue have demonstrated moderate inhibitory activity against alpha-D-mannosidase and yeast alpha-D-glucosidase. [] This finding underscores the potential of these derivatives as tools for studying enzyme activity and developing potential therapeutic agents.
Q3: Have there been any unexpected reactions observed with 1,6-Anhydro-beta-D-mannopyranose derivatives in organic synthesis?
A3: Interestingly, an unexpected regioselectivity was observed during the Rh-catalyzed sulfamate ester cyclization of 1,6-Anhydro-beta-D-mannopyranose derivatives. Instead of the anticipated six-membered oxathiazinane products, the reaction yielded five-membered sulfamidates, indicating a preference for beta-C-H amination over the typical gamma-C-H insertion. [] This unusual selectivity highlights the importance of considering three-dimensional structures and electronic factors when designing synthetic strategies involving these derivatives.
Q4: Can 1,6-Anhydro-beta-D-mannopyranose be generated unintentionally during carbohydrate analysis?
A4: Yes, it's crucial to be aware that 2,3,4-tri-O-acetyl-1,6-anhydro-beta-D-mannopyranose can form as an artifact during carbohydrate analysis. [] This underscores the importance of careful method validation and interpretation of results in carbohydrate research to avoid misidentification and ensure accurate data interpretation.
Avertissement et informations sur les produits de recherche in vitro
Veuillez noter que tous les articles et informations sur les produits présentés sur BenchChem sont destinés uniquement à des fins informatives. Les produits disponibles à l'achat sur BenchChem sont spécifiquement conçus pour des études in vitro, qui sont réalisées en dehors des organismes vivants. Les études in vitro, dérivées du terme latin "in verre", impliquent des expériences réalisées dans des environnements de laboratoire contrôlés à l'aide de cellules ou de tissus. Il est important de noter que ces produits ne sont pas classés comme médicaments et n'ont pas reçu l'approbation de la FDA pour la prévention, le traitement ou la guérison de toute condition médicale, affection ou maladie. Nous devons souligner que toute forme d'introduction corporelle de ces produits chez les humains ou les animaux est strictement interdite par la loi. Il est essentiel de respecter ces directives pour assurer la conformité aux normes légales et éthiques en matière de recherche et d'expérimentation.