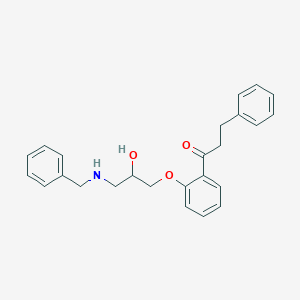
N-Depropyl N-Benzyl Propafenone
- Cliquez sur DEMANDE RAPIDE pour recevoir un devis de notre équipe d'experts.
- Avec des produits de qualité à un prix COMPÉTITIF, vous pouvez vous concentrer davantage sur votre recherche.
Vue d'ensemble
Description
N-Depropyl N-Benzyl Propafenone is a chemical compound with the molecular formula C25H27NO3 . It is used in the preparation of Propafenone analogues as antimalarial agents . The compound is also a metabolite of Propafenone .
Molecular Structure Analysis
The molecular structure of this compound consists of 25 carbon atoms, 27 hydrogen atoms, and 3 oxygen atoms . The IUPAC name for this compound is 1-[2-[3-(benzylamino)-2-hydroxypropoxy]phenyl]-3-phenylpropan-1-one .Physical and Chemical Properties Analysis
This compound has a molecular weight of 389.5 g/mol . It has a computed XLogP3-AA value of 3.9, indicating its lipophilicity . The compound has 2 hydrogen bond donors and 4 hydrogen bond acceptors . It also has a rotatable bond count of 11 . The topological polar surface area is 58.6 Ų .Mécanisme D'action
While the specific mechanism of action for N-Depropyl N-Benzyl Propafenone is not available, Propafenone, a related compound, is a class 1c antiarrhythmic agent which possesses local anesthetic properties, blocks the fast inward sodium current, and slows the rate of increase of the action potential .
Propriétés
IUPAC Name |
1-[2-[3-(benzylamino)-2-hydroxypropoxy]phenyl]-3-phenylpropan-1-one |
Source
|
---|---|---|
Source | PubChem | |
URL | https://pubchem.ncbi.nlm.nih.gov | |
Description | Data deposited in or computed by PubChem | |
InChI |
InChI=1S/C25H27NO3/c27-22(18-26-17-21-11-5-2-6-12-21)19-29-25-14-8-7-13-23(25)24(28)16-15-20-9-3-1-4-10-20/h1-14,22,26-27H,15-19H2 |
Source
|
Source | PubChem | |
URL | https://pubchem.ncbi.nlm.nih.gov | |
Description | Data deposited in or computed by PubChem | |
InChI Key |
LKPMNSBIUSAYFC-UHFFFAOYSA-N |
Source
|
Source | PubChem | |
URL | https://pubchem.ncbi.nlm.nih.gov | |
Description | Data deposited in or computed by PubChem | |
Canonical SMILES |
C1=CC=C(C=C1)CCC(=O)C2=CC=CC=C2OCC(CNCC3=CC=CC=C3)O |
Source
|
Source | PubChem | |
URL | https://pubchem.ncbi.nlm.nih.gov | |
Description | Data deposited in or computed by PubChem | |
Molecular Formula |
C25H27NO3 |
Source
|
Source | PubChem | |
URL | https://pubchem.ncbi.nlm.nih.gov | |
Description | Data deposited in or computed by PubChem | |
Molecular Weight |
389.5 g/mol |
Source
|
Source | PubChem | |
URL | https://pubchem.ncbi.nlm.nih.gov | |
Description | Data deposited in or computed by PubChem | |
Avertissement et informations sur les produits de recherche in vitro
Veuillez noter que tous les articles et informations sur les produits présentés sur BenchChem sont destinés uniquement à des fins informatives. Les produits disponibles à l'achat sur BenchChem sont spécifiquement conçus pour des études in vitro, qui sont réalisées en dehors des organismes vivants. Les études in vitro, dérivées du terme latin "in verre", impliquent des expériences réalisées dans des environnements de laboratoire contrôlés à l'aide de cellules ou de tissus. Il est important de noter que ces produits ne sont pas classés comme médicaments et n'ont pas reçu l'approbation de la FDA pour la prévention, le traitement ou la guérison de toute condition médicale, affection ou maladie. Nous devons souligner que toute forme d'introduction corporelle de ces produits chez les humains ou les animaux est strictement interdite par la loi. Il est essentiel de respecter ces directives pour assurer la conformité aux normes légales et éthiques en matière de recherche et d'expérimentation.