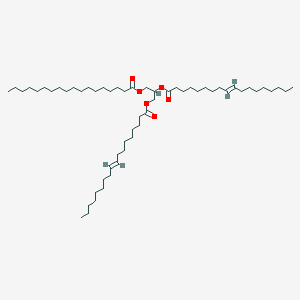
Glycerol 1,2-di-(9Z-octadecenoate) 3-octadecanoate
Vue d'ensemble
Description
1,2-Dielaidoyl-3-Stearoyl-rac-glycerol is a triacylglycerol composed of elaidic acid at the sn-1 and sn-2 positions, and stearic acid at the sn-3 position . This compound is a type of glyceride, which is a significant class of lipids found in biological systems.
Applications De Recherche Scientifique
1,2-Dielaidoyl-3-Stearoyl-rac-glycerol has several scientific research applications:
Chemistry: Used as a model compound to study lipid oxidation and hydrolysis reactions.
Biology: Investigated for its role in cellular lipid metabolism and signaling pathways.
Medicine: Studied for its potential effects on lipid-related disorders and its use in drug delivery systems.
Industry: Utilized in the formulation of cosmetics and food products due to its emollient properties.
Méthodes De Préparation
Synthetic Routes and Reaction Conditions
1,2-Dielaidoyl-3-Stearoyl-rac-glycerol can be synthesized through esterification reactions involving glycerol and the respective fatty acids. The reaction typically involves the use of catalysts such as sulfuric acid or p-toluenesulfonic acid under reflux conditions . The reaction mixture is then purified using techniques such as column chromatography to isolate the desired product.
Industrial Production Methods
In industrial settings, the production of 1,2-Dielaidoyl-3-Stearoyl-rac-glycerol involves large-scale esterification processes. These processes often use continuous flow reactors to maintain optimal reaction conditions and maximize yield. The product is then subjected to various purification steps, including distillation and crystallization, to ensure high purity .
Analyse Des Réactions Chimiques
Types of Reactions
1,2-Dielaidoyl-3-Stearoyl-rac-glycerol undergoes several types of chemical reactions, including:
Oxidation: This reaction can lead to the formation of peroxides and other oxidative degradation products.
Hydrolysis: In the presence of water and enzymes such as lipases, the compound can be hydrolyzed to release free fatty acids and glycerol.
Transesterification: This reaction involves the exchange of ester groups between different glycerides or between glycerides and alcohols.
Common Reagents and Conditions
Oxidation: Common oxidizing agents include potassium permanganate and hydrogen peroxide.
Hydrolysis: Enzymatic hydrolysis typically uses lipases under mild aqueous conditions.
Transesterification: Catalysts such as sodium methoxide or sulfuric acid are commonly used under reflux conditions.
Major Products Formed
Oxidation: Peroxides and aldehydes.
Hydrolysis: Free fatty acids (elaidic acid and stearic acid) and glycerol.
Transesterification: New glycerides and alcohols.
Mécanisme D'action
The mechanism of action of 1,2-Dielaidoyl-3-Stearoyl-rac-glycerol involves its interaction with lipid membranes and enzymes. The compound can integrate into lipid bilayers, affecting membrane fluidity and permeability. It also serves as a substrate for lipases, which hydrolyze the ester bonds to release free fatty acids and glycerol. These free fatty acids can then participate in various metabolic pathways, influencing cellular functions .
Comparaison Avec Des Composés Similaires
Similar Compounds
1,2-Dioleoyl-3-Stearoyl-rac-glycerol: Contains oleic acid instead of elaidic acid at the sn-1 and sn-2 positions.
1,2-Distearoyl-3-Linoleoyl-rac-glycerol: Contains stearic acid at the sn-1 and sn-2 positions and linoleic acid at the sn-3 position.
Uniqueness
1,2-Dielaidoyl-3-Stearoyl-rac-glycerol is unique due to the presence of elaidic acid, a trans fatty acid, at the sn-1 and sn-2 positions. This configuration imparts distinct physical and chemical properties, such as higher melting points and different reactivity compared to its cis counterparts .
Propriétés
IUPAC Name |
2,3-bis[[(E)-octadec-9-enoyl]oxy]propyl octadecanoate | |
---|---|---|
Source | PubChem | |
URL | https://pubchem.ncbi.nlm.nih.gov | |
Description | Data deposited in or computed by PubChem | |
InChI |
InChI=1S/C57H106O6/c1-4-7-10-13-16-19-22-25-28-31-34-37-40-43-46-49-55(58)61-52-54(63-57(60)51-48-45-42-39-36-33-30-27-24-21-18-15-12-9-6-3)53-62-56(59)50-47-44-41-38-35-32-29-26-23-20-17-14-11-8-5-2/h25,27-28,30,54H,4-24,26,29,31-53H2,1-3H3/b28-25+,30-27+ | |
Source | PubChem | |
URL | https://pubchem.ncbi.nlm.nih.gov | |
Description | Data deposited in or computed by PubChem | |
InChI Key |
RYNHWWNZNIGDAQ-WGSDILPMSA-N | |
Source | PubChem | |
URL | https://pubchem.ncbi.nlm.nih.gov | |
Description | Data deposited in or computed by PubChem | |
Canonical SMILES |
CCCCCCCCCCCCCCCCCC(=O)OCC(COC(=O)CCCCCCCC=CCCCCCCCC)OC(=O)CCCCCCCC=CCCCCCCCC | |
Source | PubChem | |
URL | https://pubchem.ncbi.nlm.nih.gov | |
Description | Data deposited in or computed by PubChem | |
Isomeric SMILES |
CCCCCCCCCCCCCCCCCC(=O)OCC(COC(=O)CCCCCCC/C=C/CCCCCCCC)OC(=O)CCCCCCC/C=C/CCCCCCCC | |
Source | PubChem | |
URL | https://pubchem.ncbi.nlm.nih.gov | |
Description | Data deposited in or computed by PubChem | |
Molecular Formula |
C57H106O6 | |
Source | PubChem | |
URL | https://pubchem.ncbi.nlm.nih.gov | |
Description | Data deposited in or computed by PubChem | |
Molecular Weight |
887.4 g/mol | |
Source | PubChem | |
URL | https://pubchem.ncbi.nlm.nih.gov | |
Description | Data deposited in or computed by PubChem | |
Physical Description |
Solid | |
Record name | Glycerol 1,2-di-(9Z-octadecenoate) 3-octadecanoate | |
Source | Human Metabolome Database (HMDB) | |
URL | http://www.hmdb.ca/metabolites/HMDB0031144 | |
Description | The Human Metabolome Database (HMDB) is a freely available electronic database containing detailed information about small molecule metabolites found in the human body. | |
Explanation | HMDB is offered to the public as a freely available resource. Use and re-distribution of the data, in whole or in part, for commercial purposes requires explicit permission of the authors and explicit acknowledgment of the source material (HMDB) and the original publication (see the HMDB citing page). We ask that users who download significant portions of the database cite the HMDB paper in any resulting publications. | |
CAS RN |
2410-28-8 | |
Record name | Glycerol 1,2-di-(9Z-octadecenoate) 3-octadecanoate | |
Source | Human Metabolome Database (HMDB) | |
URL | http://www.hmdb.ca/metabolites/HMDB0031144 | |
Description | The Human Metabolome Database (HMDB) is a freely available electronic database containing detailed information about small molecule metabolites found in the human body. | |
Explanation | HMDB is offered to the public as a freely available resource. Use and re-distribution of the data, in whole or in part, for commercial purposes requires explicit permission of the authors and explicit acknowledgment of the source material (HMDB) and the original publication (see the HMDB citing page). We ask that users who download significant portions of the database cite the HMDB paper in any resulting publications. | |
Melting Point |
22.5 - 23.5 °C | |
Record name | Glycerol 1,2-di-(9Z-octadecenoate) 3-octadecanoate | |
Source | Human Metabolome Database (HMDB) | |
URL | http://www.hmdb.ca/metabolites/HMDB0031144 | |
Description | The Human Metabolome Database (HMDB) is a freely available electronic database containing detailed information about small molecule metabolites found in the human body. | |
Explanation | HMDB is offered to the public as a freely available resource. Use and re-distribution of the data, in whole or in part, for commercial purposes requires explicit permission of the authors and explicit acknowledgment of the source material (HMDB) and the original publication (see the HMDB citing page). We ask that users who download significant portions of the database cite the HMDB paper in any resulting publications. | |
Retrosynthesis Analysis
AI-Powered Synthesis Planning: Our tool employs the Template_relevance Pistachio, Template_relevance Bkms_metabolic, Template_relevance Pistachio_ringbreaker, Template_relevance Reaxys, Template_relevance Reaxys_biocatalysis model, leveraging a vast database of chemical reactions to predict feasible synthetic routes.
One-Step Synthesis Focus: Specifically designed for one-step synthesis, it provides concise and direct routes for your target compounds, streamlining the synthesis process.
Accurate Predictions: Utilizing the extensive PISTACHIO, BKMS_METABOLIC, PISTACHIO_RINGBREAKER, REAXYS, REAXYS_BIOCATALYSIS database, our tool offers high-accuracy predictions, reflecting the latest in chemical research and data.
Strategy Settings
Precursor scoring | Relevance Heuristic |
---|---|
Min. plausibility | 0.01 |
Model | Template_relevance |
Template Set | Pistachio/Bkms_metabolic/Pistachio_ringbreaker/Reaxys/Reaxys_biocatalysis |
Top-N result to add to graph | 6 |
Feasible Synthetic Routes
Avertissement et informations sur les produits de recherche in vitro
Veuillez noter que tous les articles et informations sur les produits présentés sur BenchChem sont destinés uniquement à des fins informatives. Les produits disponibles à l'achat sur BenchChem sont spécifiquement conçus pour des études in vitro, qui sont réalisées en dehors des organismes vivants. Les études in vitro, dérivées du terme latin "in verre", impliquent des expériences réalisées dans des environnements de laboratoire contrôlés à l'aide de cellules ou de tissus. Il est important de noter que ces produits ne sont pas classés comme médicaments et n'ont pas reçu l'approbation de la FDA pour la prévention, le traitement ou la guérison de toute condition médicale, affection ou maladie. Nous devons souligner que toute forme d'introduction corporelle de ces produits chez les humains ou les animaux est strictement interdite par la loi. Il est essentiel de respecter ces directives pour assurer la conformité aux normes légales et éthiques en matière de recherche et d'expérimentation.