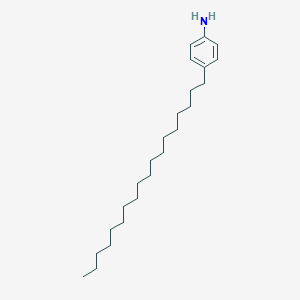
4-Octadecylaniline
Vue d'ensemble
Description
4-Octadecylaniline is an organic compound with the chemical formula C24H43N . It is characterized by a long alkyl chain attached to an aniline group, making it a unique molecule with both hydrophobic and hydrophilic properties. This compound appears as a white or yellowish crystalline solid and is soluble in organic solvents such as alcohols, ethers, and aromatic hydrocarbons, but insoluble in water .
Applications De Recherche Scientifique
4-Octadecylaniline has a wide range of applications in scientific research:
Biology: The compound’s amphiphilic nature makes it useful in the formulation of surfactants and emulsifiers for biological studies.
Medicine: It is explored for its potential use in drug delivery systems due to its ability to interact with biological membranes.
Industry: this compound is used as a rubber additive to improve tensile properties, wear resistance, and aging resistance.
Mécanisme D'action
Target of Action
This compound is a long-chain aniline derivative, and its interactions with biological targets are likely to be influenced by its lipophilic alkyl chain and the presence of the aniline group .
Mode of Action
It has been used in the fabrication of alternate-layer langmuir-blodgett (lb) films, suggesting that it may interact with lipid membranes or other hydrophobic targets . The aniline group could potentially undergo various chemical reactions, contributing to its biological activity.
Pharmacokinetics
The ADME (Absorption, Distribution, Metabolism, and Excretion) properties of 4-Octadecylaniline are not well-studied. Its long alkyl chain suggests it may have good lipid solubility, potentially influencing its absorption and distribution. The aniline group could be subject to metabolic transformations .
Result of Action
Its use in the fabrication of LB films suggests it may influence membrane properties or functions
Action Environment
Environmental factors such as temperature, pH, and the presence of other molecules could influence the action, efficacy, and stability of this compound. Its long alkyl chain might interact with other hydrophobic substances, and its aniline group could be sensitive to changes in pH .
Méthodes De Préparation
Synthetic Routes and Reaction Conditions: 4-Octadecylaniline can be synthesized through the reaction of aniline with octadecyl halides under basic conditions. The reaction typically involves heating aniline with octadecyl bromide in the presence of a base such as sodium hydroxide or potassium carbonate. The reaction is carried out in an organic solvent like toluene or ethanol, and the product is purified through recrystallization .
Industrial Production Methods: In industrial settings, the production of this compound may involve the use of continuous flow reactors to ensure consistent quality and yield. The reaction conditions are optimized to maximize the conversion of reactants to the desired product while minimizing by-products. The use of catalysts and advanced purification techniques such as distillation and chromatography may also be employed to enhance the efficiency of the process .
Analyse Des Réactions Chimiques
Types of Reactions: 4-Octadecylaniline undergoes various chemical reactions, including:
Oxidation: It can be oxidized to form corresponding nitro compounds or quinones.
Reduction: The nitro derivatives can be reduced back to the amine.
Substitution: The aniline group can undergo electrophilic substitution reactions, such as nitration, sulfonation, and halogenation.
Common Reagents and Conditions:
Oxidation: Common oxidizing agents include potassium permanganate and chromium trioxide.
Reduction: Reducing agents like hydrogen gas in the presence of a palladium catalyst or sodium borohydride are used.
Substitution: Reagents such as nitric acid for nitration, sulfuric acid for sulfonation, and halogens like chlorine or bromine for halogenation.
Major Products Formed:
Oxidation: Nitro derivatives and quinones.
Reduction: Primary amines.
Substitution: Nitroanilines, sulfonated anilines, and halogenated anilines.
Comparaison Avec Des Composés Similaires
- 4-Octylaniline
- 4-Tetradecylaniline
- 4-Hexadecylaniline
Comparison: 4-Octadecylaniline is unique due to its longer alkyl chain, which imparts distinct hydrophobic properties compared to its shorter-chain analogs. This makes it particularly useful in applications requiring strong hydrophobic interactions, such as in the formulation of surfactants and emulsifiers .
Activité Biologique
4-Octadecylaniline is a long-chain aniline derivative that has garnered attention for its diverse biological activities and applications in various fields, including chemistry, biology, and medicine. This article explores its biological activity, mechanisms of action, pharmacokinetics, and potential applications based on current research findings.
Chemical Structure and Properties
This compound is characterized by its long alkyl chain (C18) attached to an aniline group. This structure imparts amphiphilic properties to the compound, making it suitable for various applications in surfactants and emulsifiers. Its chemical formula is C19H39N, and it has a molecular weight of 281.5 g/mol.
Biological Activity
The biological activity of this compound can be attributed to its interactions with cellular membranes and proteins due to its lipophilic nature. Key areas of investigation include:
- Cell Membrane Interaction : The compound's long alkyl chain allows it to integrate into lipid bilayers, potentially influencing membrane fluidity and permeability.
- Drug Delivery Systems : Its ability to interact with biological membranes makes it a candidate for drug delivery applications, facilitating the transport of therapeutic agents across cellular barriers.
- Antimicrobial Activity : Preliminary studies suggest that this compound may exhibit antimicrobial properties, although specific mechanisms remain to be elucidated.
Target of Action
This compound's interactions are likely influenced by both the hydrophobic alkyl chain and the polar amine group. This duality allows for versatile interactions with various biological targets, including:
- Lipid Membranes : The compound can alter membrane properties, potentially affecting the function of membrane-bound proteins.
- Protein Interactions : The aniline moiety may engage in hydrogen bonding or hydrophobic interactions with amino acid residues in proteins.
Mode of Action
The mode of action involves integration into lipid bilayers, which can disrupt membrane integrity or alter signaling pathways. Additionally, it may undergo metabolic transformations that could influence its biological effects.
Pharmacokinetics
The pharmacokinetic profile of this compound has not been extensively studied; however, its long alkyl chain suggests favorable lipid solubility, which may enhance absorption and distribution within biological systems. The Absorption, Distribution, Metabolism, and Excretion (ADME) properties are critical for understanding its potential therapeutic applications.
Parameter | Description |
---|---|
Absorption | Likely high due to lipophilicity; specific studies needed |
Distribution | Predicted to distribute widely in lipid-rich tissues |
Metabolism | Potential for oxidative metabolism via the aniline group |
Excretion | Expected to be excreted primarily via urine after metabolic conversion |
Synthesis and Characterization
Research has demonstrated that this compound can be synthesized through various methods, including oxidative polymerization. Studies have shown that modifying polyaniline with octadecyl chains significantly alters its electrical properties and morphology .
Applications in Langmuir-Blodgett Films
This compound has been utilized in the fabrication of Langmuir-Blodgett (LB) films. These films are instrumental in studying molecular interactions at interfaces and have potential applications in biosensors and electronic devices.
Safety Profile
Despite its promising applications, this compound is classified as a skin irritant (Category 2) and eye irritant (Category 2A). It may also cause respiratory irritation upon exposure. Safety data sheets should be consulted before handling this compound.
Propriétés
IUPAC Name |
4-octadecylaniline | |
---|---|---|
Source | PubChem | |
URL | https://pubchem.ncbi.nlm.nih.gov | |
Description | Data deposited in or computed by PubChem | |
InChI |
InChI=1S/C24H43N/c1-2-3-4-5-6-7-8-9-10-11-12-13-14-15-16-17-18-23-19-21-24(25)22-20-23/h19-22H,2-18,25H2,1H3 | |
Source | PubChem | |
URL | https://pubchem.ncbi.nlm.nih.gov | |
Description | Data deposited in or computed by PubChem | |
InChI Key |
CCRTYBGROAGVGK-UHFFFAOYSA-N | |
Source | PubChem | |
URL | https://pubchem.ncbi.nlm.nih.gov | |
Description | Data deposited in or computed by PubChem | |
Canonical SMILES |
CCCCCCCCCCCCCCCCCCC1=CC=C(C=C1)N | |
Source | PubChem | |
URL | https://pubchem.ncbi.nlm.nih.gov | |
Description | Data deposited in or computed by PubChem | |
Molecular Formula |
C24H43N | |
Source | PubChem | |
URL | https://pubchem.ncbi.nlm.nih.gov | |
Description | Data deposited in or computed by PubChem | |
DSSTOX Substance ID |
DTXSID10393672 | |
Record name | 4-Octadecylaniline | |
Source | EPA DSSTox | |
URL | https://comptox.epa.gov/dashboard/DTXSID10393672 | |
Description | DSSTox provides a high quality public chemistry resource for supporting improved predictive toxicology. | |
Molecular Weight |
345.6 g/mol | |
Source | PubChem | |
URL | https://pubchem.ncbi.nlm.nih.gov | |
Description | Data deposited in or computed by PubChem | |
CAS No. |
114235-67-5 | |
Record name | 4-Octadecylaniline | |
Source | EPA DSSTox | |
URL | https://comptox.epa.gov/dashboard/DTXSID10393672 | |
Description | DSSTox provides a high quality public chemistry resource for supporting improved predictive toxicology. | |
Retrosynthesis Analysis
AI-Powered Synthesis Planning: Our tool employs the Template_relevance Pistachio, Template_relevance Bkms_metabolic, Template_relevance Pistachio_ringbreaker, Template_relevance Reaxys, Template_relevance Reaxys_biocatalysis model, leveraging a vast database of chemical reactions to predict feasible synthetic routes.
One-Step Synthesis Focus: Specifically designed for one-step synthesis, it provides concise and direct routes for your target compounds, streamlining the synthesis process.
Accurate Predictions: Utilizing the extensive PISTACHIO, BKMS_METABOLIC, PISTACHIO_RINGBREAKER, REAXYS, REAXYS_BIOCATALYSIS database, our tool offers high-accuracy predictions, reflecting the latest in chemical research and data.
Strategy Settings
Precursor scoring | Relevance Heuristic |
---|---|
Min. plausibility | 0.01 |
Model | Template_relevance |
Template Set | Pistachio/Bkms_metabolic/Pistachio_ringbreaker/Reaxys/Reaxys_biocatalysis |
Top-N result to add to graph | 6 |
Feasible Synthetic Routes
Q1: What makes 4-octadecylaniline suitable for creating pyroelectric Langmuir-Blodgett films?
A1: this compound, when alternated with 22-tricosenoic acid in LB films, contributes to a high pyroelectric coefficient. This is attributed to the specific inter-layer bonding between these molecules, as revealed by infrared spectroscopy []. The long alkyl chain in this compound likely promotes organized packing within the film, while the terminal amine group can interact with the carboxylic acid group of 22-tricosenoic acid, potentially through hydrogen bonding, influencing the overall dipole moment and thus pyroelectric properties.
Q2: How does the choice of substrate affect the pyroelectric performance of LB films containing this compound?
A2: The research indicates that the substrate can negatively impact the responsivity of LB film devices. Furthermore, studies using different substrate materials revealed a significant piezoelectrically induced secondary effect on the overall pyroelectric coefficient, especially at temperatures around 250 K []. This suggests that mechanical strain at the film-substrate interface, influenced by substrate properties, can impact the film's electrical response to temperature changes.
Avertissement et informations sur les produits de recherche in vitro
Veuillez noter que tous les articles et informations sur les produits présentés sur BenchChem sont destinés uniquement à des fins informatives. Les produits disponibles à l'achat sur BenchChem sont spécifiquement conçus pour des études in vitro, qui sont réalisées en dehors des organismes vivants. Les études in vitro, dérivées du terme latin "in verre", impliquent des expériences réalisées dans des environnements de laboratoire contrôlés à l'aide de cellules ou de tissus. Il est important de noter que ces produits ne sont pas classés comme médicaments et n'ont pas reçu l'approbation de la FDA pour la prévention, le traitement ou la guérison de toute condition médicale, affection ou maladie. Nous devons souligner que toute forme d'introduction corporelle de ces produits chez les humains ou les animaux est strictement interdite par la loi. Il est essentiel de respecter ces directives pour assurer la conformité aux normes légales et éthiques en matière de recherche et d'expérimentation.