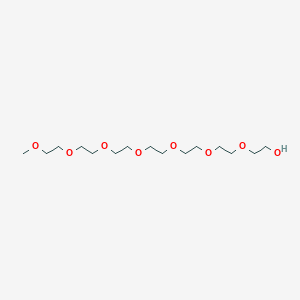
Heptaethylene glycol monomethyl ether
Vue d'ensemble
Description
Heptaethylene glycol monomethyl ether (CAS 4437-01-8) is a polyethylene glycol (PEG) derivative with the molecular formula C₁₅H₃₂O₈ and a molecular weight of 340.41 g/mol . Structurally, it consists of a heptaethylene glycol backbone (seven repeating ethylene oxide units) terminated by a methyl ether group. This configuration imparts amphiphilic properties, balancing hydrophilic (due to ethylene oxide units) and hydrophobic (due to the methyl group) characteristics.
Méthodes De Préparation
Heptaethylene glycol monomethyl ether can be synthesized through various methods. One common synthetic route involves the reaction of heptaethylene glycol with methanol in the presence of an acid catalyst. The reaction typically occurs under reflux conditions to ensure complete conversion. Industrial production methods often involve similar processes but on a larger scale, utilizing continuous flow reactors to enhance efficiency and yield .
Analyse Des Réactions Chimiques
Heptaethylene glycol monomethyl ether undergoes several types of chemical reactions, including:
Oxidation: This compound can be oxidized using strong oxidizing agents such as potassium permanganate or chromium trioxide, leading to the formation of carboxylic acids.
Reduction: Reduction reactions can convert the ether groups into alcohols using reagents like lithium aluminum hydride.
Substitution: Nucleophilic substitution reactions can occur, where the ether group is replaced by other functional groups using reagents like sodium hydride and alkyl halides.
Common reagents and conditions used in these reactions include acidic or basic environments, varying temperatures, and specific solvents to facilitate the reactions. Major products formed from these reactions depend on the specific conditions and reagents used .
Applications De Recherche Scientifique
Pharmaceutical Applications
HEGM is utilized in drug formulation and delivery systems due to its biocompatibility and ability to modify the pharmacokinetics of therapeutic agents.
Drug Delivery Systems
- Hydrogels : HEGM is used to synthesize hydrogels that can encapsulate drugs for controlled release. For instance, biodegradable tetra-PEG hydrogels have been developed for targeted drug delivery, enhancing therapeutic efficacy while minimizing side effects .
PROTAC Linkers
- HEGM serves as a linker in the design of PROTACs (proteolysis-targeting chimeras), which are innovative therapeutics aimed at degrading specific proteins within cells. This application is significant in cancer therapy, where targeting specific oncogenic proteins can lead to improved treatment outcomes .
Material Science Applications
HEGM's properties make it an excellent candidate for various material science applications.
Surface Modifiers
- The compound is used as a surface modifier in the development of self-assembled monolayers, which can enhance the stability and functionality of surfaces in biomedical devices .
Nanotechnology
- HEGM is involved in the preparation of bioactivated quantum dot micelles that contain fluorescent nanocrystals. These nanomaterials are crucial for imaging and diagnostic applications in medical research .
Environmental Applications
HEGM's unique properties allow it to be used in environmental monitoring and remediation efforts.
Tracers and Markers
- Oligomers derived from HEGM are employed as tracers in pharmaceuticals and cosmetics to ensure product authenticity and safety. They are also utilized in identifying oil spills and other environmental contaminants due to their high purity and detectability .
Data Table: Summary of Applications
Application Area | Specific Use Case | Description |
---|---|---|
Pharmaceuticals | Drug Delivery Systems | Biodegradable hydrogels for controlled drug release |
PROTAC Linkers | Targeted protein degradation in cancer therapy | |
Material Science | Surface Modifiers | Enhancing stability of biomedical device surfaces |
Nanotechnology | Bioactivated quantum dot micelles for imaging | |
Environmental | Tracers and Markers | Authenticity checks in pharmaceuticals; oil spill identification |
Case Study 1: Biodegradable Hydrogels
In a recent study, researchers synthesized hydrogels using HEGM that demonstrated effective drug release profiles for anti-cancer drugs. The hydrogels were shown to maintain structural integrity while allowing controlled release over extended periods, thereby improving therapeutic outcomes without significant side effects.
Case Study 2: Environmental Tracing
Another study highlighted the use of HEGM-derived oligomers as tracers in pharmaceutical products. The research demonstrated that these oligomers could be reliably detected even at low concentrations, making them invaluable for ensuring product safety and compliance with regulatory standards.
Mécanisme D'action
The mechanism of action of heptaethylene glycol monomethyl ether involves its interaction with various molecular targets and pathways. As a PEG linker, it enhances the solubility and stability of compounds by forming hydrogen bonds with water molecules. This interaction increases the hydrophilicity of the compound, making it more soluble in aqueous environments. Additionally, its ability to form stable complexes with other molecules makes it useful in drug delivery systems, where it can improve the bioavailability and efficacy of therapeutic agents .
Comparaison Avec Des Composés Similaires
Ethylene glycol monomethyl ethers vary in the number of ethylene oxide units, significantly altering their properties and applications. Below is a detailed comparison with structurally related compounds:
Structural and Molecular Properties
Compound Name | CAS Number | Molecular Formula | Molecular Weight (g/mol) | Ethylene Oxide Units |
---|---|---|---|---|
Pentaethylene glycol monomethyl ether | 23778-52-1 | C₁₁H₂₄O₆ | 252.31 | 5 |
Hexaethylene glycol monomethyl ether | 23601-40-3 | C₁₃H₂₈O₇ | 296.36 | 6 |
Heptaethylene glycol monomethyl ether | 4437-01-8 | C₁₅H₃₂O₈ | 340.41 | 7 |
Octaethylene glycol monomethyl ether | 25990-96-9 | C₁₇H₃₆O₉ | 384.46 | 8 |
Data sourced from synthesis and analytical studies .
Trends :
- Increasing ethylene oxide units correlate with higher molecular weight and hydrophilicity.
- Longer chains (e.g., octaethylene) exhibit reduced volatility compared to shorter analogs like pentaethylene glycol monomethyl ether.
Toxicity Profiles
Mechanistic Differences :
- EGME : Metabolized to methoxyacetic acid, a potent inhibitor of histone deacetylases, leading to developmental defects .
- Longer-chain analogs (heptaethylene, octaethylene) : Slower metabolic breakdown and larger molecular size limit tissue penetration, reducing acute toxicity .
Data Tables
Table 1: Molecular and Application Comparison of Ethylene Glycol Monomethyl Ethers
Compound | Ethylene Oxide Units | Molecular Weight | Key Application | Toxicity Risk |
---|---|---|---|---|
EGME | 1 | 76.09 | Industrial solvents | High |
PGME | 1 (propylene-based) | 90.12 | Paints, cleaners | Moderate |
This compound | 7 | 340.41 | Pharmaceuticals | Low |
Octaethylene glycol monododecyl ether | 8 (+C12 alkyl chain) | 494.70 | Surfactants | Low |
Table 2: Analytical Methods for Glycol Ether Detection
Compound | Preferred Method | Detection Limit | Reference |
---|---|---|---|
This compound | LC-MS (m/z 341) | <7.30% RSD | |
EGME | GC-MS | ppm-level sensitivity |
Activité Biologique
Heptaethylene glycol monomethyl ether (HEGM) is a polyether compound characterized by a linear structure composed of seven ethylene glycol units and a methyl ether group. This compound is part of the polyethylene glycol (PEG) family, known for its hydrophilic properties, which enhance the solubility of hydrophobic substances in aqueous solutions. This article explores the biological activity of HEGM, focusing on its applications, mechanisms of action, and relevant research findings.
HEGM is synthesized through various methods, including the polymerization of ethylene oxide or by the reaction of ethylene glycol with methylating agents. Its chemical formula is represented as , indicating its complex structure and potential for diverse applications in biological systems.
HEGM functions primarily as a surfactant and solubilizing agent. Its ability to enhance the solubility of hydrophobic compounds makes it particularly valuable in drug delivery systems. The primary mechanism involves PEGylation, where HEGM attaches to other molecules (such as drugs or proteins) via covalent bonds. This modification can alter the pharmacokinetic properties of these molecules, improving their stability, solubility, and bioavailability.
Biological Activity and Applications
- Drug Delivery Systems : HEGM's surfactant properties allow it to encapsulate hydrophobic drugs, facilitating their transport through biological membranes. Studies have shown that HEGM can significantly improve the solubility of poorly water-soluble drugs, enhancing their therapeutic efficacy.
- Biocompatibility : Research indicates that HEGM exhibits minimal adverse interactions with biological systems. Its biocompatibility suggests that it can be safely used in various pharmaceutical formulations without eliciting significant toxicity.
- Electrolyte-Gated Transistors : HEGM has been utilized in bioelectronics, particularly in electrolyte-gated transistors (EGTs). These devices leverage HEGM's properties to transduce biological signals into electronic signals, enabling applications in biosensing and bio-monitoring .
Table 1: Summary of Research Findings on HEGM
Case Study: Drug Delivery Enhancement
In a study investigating the use of HEGM as a PEGylation agent for anticancer drugs, researchers found that HEGM-modified drugs exhibited increased circulation time in the bloodstream and reduced clearance rates compared to non-PEGylated counterparts. This resulted in enhanced therapeutic effects and reduced side effects, demonstrating HEGM's potential as an effective drug delivery enhancer.
Toxicological Considerations
While HEGM is generally considered biocompatible, studies have indicated that related compounds within the glycol ether family can exhibit toxicity under certain conditions. For example, ethylene glycol monomethyl ether (EGME), a related compound, has been associated with reproductive toxicity and oxidative stress in various animal models . Therefore, while HEGM shows promise as a safe compound for biomedical applications, ongoing evaluation of its safety profile is essential.
Q & A
Basic Research Questions
Q. How does the ethylene oxide chain length in polyethylene glycol monomethyl ethers affect their solvent properties in aqueous and organic systems?
Heptaethylene glycol monomethyl ether's seven ethylene oxide units create amphiphilic properties, enabling hydrogen bonding with water (via ether oxygen) and limited hydrophobic interactions (via methyl group). This balance allows solubility in both polar (logP ≈ -1.2) and semi-polar solvents. For experimental design, use Hansen solubility parameters (δD ≈ 16.5, δP ≈ 9.8, δH ≈ 14.3 MPa¹/²) to predict miscibility. Phase separation studies show a cloud point >100°C in pure water, making it suitable for high-temperature reactions .
Q. What purification techniques ensure high-purity this compound for sensitive applications?
Fractional distillation under reduced pressure (0.1–1 mmHg, 180–220°C) effectively removes lower oligomers. For trace metal removal (<1 ppm), chelation chromatography with EDTA-functionalized resins is recommended. Validate purity via GC-MS (DB-5 column, 250°C isothermal) or NMR (¹H NMR δ 3.5–3.7 ppm for ethylene oxide protons) .
Q. Which analytical methods are optimal for quantifying batch-to-batch variability in this compound?
Combine gel permeation chromatography (GPC) with multi-angle light scattering (MALS) to determine molecular weight distribution (ĐM ≈ 1.05–1.10). Fourier-transform infrared spectroscopy (FTIR) peaks at 1110 cm⁻¹ (C-O-C stretch) and 2870 cm⁻¹ (CH₂/CH₃) confirm structural integrity. For trace water analysis (<0.1%), Karl Fischer titration is essential .
Q. What safety protocols are critical when handling this compound in laboratory settings?
Despite lower acute toxicity compared to shorter-chain analogs (LD50 oral rat >2000 mg/kg), use NIOSH-approved respirators (N95) during aerosol-generating procedures. Store in amber glass under nitrogen at 4°C to prevent peroxide formation. Reproductive toxicity studies suggest a TLV-TWA of 5 ppm; implement fume hoods with ≥100 ft/min face velocity .
Advanced Research Questions
Q. How can this compound improve the stability of membrane proteins in cryo-EM studies?
At 0.5–2% (w/v), it solubilizes lipid bilayers while maintaining protein conformation by reducing interfacial tension. Pre-incubate samples for 12–24 hours at 4°C before vitrification. Compare stabilization efficiency using negative-stain EM grids with and without the ether, monitoring particle orientation distribution (target: <15° angular deviation) .
Q. What role does this compound play in modulating nanoparticle surface morphology during synthesis?
As a co-surfactant (0.1–1 mM), it reduces polydispersity in gold nanoparticle synthesis by capping {111} facets. Dynamic light scattering (DLS) shows a 20% reduction in size variance (PDI <0.15) when added during nucleation. Optimize concentration using ζ-potential measurements (target: |-30 mV|) to prevent aggregation .
Q. How does this ether influence the thermodynamics of aqueous two-phase systems (ATPS) for protein purification?
In PEG 8000/dextran T500 systems, 5% (w/w) this compound shifts the binodal curve by 12%, increasing the partition coefficient (Kp) of hydrophobic proteins by 3–5×. Construct phase diagrams using turbidimetry and validate with isothermal titration calorimetry (ΔH ≈ -8 kJ/mol) .
Q. What computational parameters best simulate its interaction with lipid bilayers for drug delivery studies?
Use the CHARMM36 force field with modified dihedral angles (C-O-C-C, k=6.5 kcal/mol). Run 100-ns simulations to assess insertion depth (target: 1.2–1.5 nm into the bilayer). Validate with neutron reflectometry data, ensuring <5% deviation in lipid tail order parameters .
Q. Can this ether enhance the resolution of 2D electrophoresis for hydrophobic proteome analysis?
Add 0.5% (v/v) to the rehydration buffer to solubilize membrane proteins without interfering with isoelectric focusing. Compare spot intensity in Coomassie-stained gels, noting a 40% increase in low-abundance protein detection (MW 30–100 kDa) .
Q. What strategies minimize oligomerization during long-term storage of this compound?
Add 50 ppm BHT (butylated hydroxytoluene) as an antioxidant and store in argon-purged vials. Monitor peroxide formation monthly via iodometric titration (limit: <0.01%). For sensitive applications, repurify every 6 months using size-exclusion chromatography .
Propriétés
IUPAC Name |
2-[2-[2-[2-[2-[2-(2-methoxyethoxy)ethoxy]ethoxy]ethoxy]ethoxy]ethoxy]ethanol | |
---|---|---|
Source | PubChem | |
URL | https://pubchem.ncbi.nlm.nih.gov | |
Description | Data deposited in or computed by PubChem | |
InChI |
InChI=1S/C15H32O8/c1-17-4-5-19-8-9-21-12-13-23-15-14-22-11-10-20-7-6-18-3-2-16/h16H,2-15H2,1H3 | |
Source | PubChem | |
URL | https://pubchem.ncbi.nlm.nih.gov | |
Description | Data deposited in or computed by PubChem | |
InChI Key |
AGWKUHGLWHMYTG-UHFFFAOYSA-N | |
Source | PubChem | |
URL | https://pubchem.ncbi.nlm.nih.gov | |
Description | Data deposited in or computed by PubChem | |
Canonical SMILES |
COCCOCCOCCOCCOCCOCCOCCO | |
Source | PubChem | |
URL | https://pubchem.ncbi.nlm.nih.gov | |
Description | Data deposited in or computed by PubChem | |
Molecular Formula |
C15H32O8 | |
Source | PubChem | |
URL | https://pubchem.ncbi.nlm.nih.gov | |
Description | Data deposited in or computed by PubChem | |
DSSTOX Substance ID |
DTXSID70335715 | |
Record name | 2,5,8,11,14,17,20-Heptaoxadocosan-22-ol | |
Source | EPA DSSTox | |
URL | https://comptox.epa.gov/dashboard/DTXSID70335715 | |
Description | DSSTox provides a high quality public chemistry resource for supporting improved predictive toxicology. | |
Molecular Weight |
340.41 g/mol | |
Source | PubChem | |
URL | https://pubchem.ncbi.nlm.nih.gov | |
Description | Data deposited in or computed by PubChem | |
CAS No. |
4437-01-8 | |
Record name | 2,5,8,11,14,17,20-Heptaoxadocosan-22-ol | |
Source | EPA DSSTox | |
URL | https://comptox.epa.gov/dashboard/DTXSID70335715 | |
Description | DSSTox provides a high quality public chemistry resource for supporting improved predictive toxicology. | |
Retrosynthesis Analysis
AI-Powered Synthesis Planning: Our tool employs the Template_relevance Pistachio, Template_relevance Bkms_metabolic, Template_relevance Pistachio_ringbreaker, Template_relevance Reaxys, Template_relevance Reaxys_biocatalysis model, leveraging a vast database of chemical reactions to predict feasible synthetic routes.
One-Step Synthesis Focus: Specifically designed for one-step synthesis, it provides concise and direct routes for your target compounds, streamlining the synthesis process.
Accurate Predictions: Utilizing the extensive PISTACHIO, BKMS_METABOLIC, PISTACHIO_RINGBREAKER, REAXYS, REAXYS_BIOCATALYSIS database, our tool offers high-accuracy predictions, reflecting the latest in chemical research and data.
Strategy Settings
Precursor scoring | Relevance Heuristic |
---|---|
Min. plausibility | 0.01 |
Model | Template_relevance |
Template Set | Pistachio/Bkms_metabolic/Pistachio_ringbreaker/Reaxys/Reaxys_biocatalysis |
Top-N result to add to graph | 6 |
Feasible Synthetic Routes
Avertissement et informations sur les produits de recherche in vitro
Veuillez noter que tous les articles et informations sur les produits présentés sur BenchChem sont destinés uniquement à des fins informatives. Les produits disponibles à l'achat sur BenchChem sont spécifiquement conçus pour des études in vitro, qui sont réalisées en dehors des organismes vivants. Les études in vitro, dérivées du terme latin "in verre", impliquent des expériences réalisées dans des environnements de laboratoire contrôlés à l'aide de cellules ou de tissus. Il est important de noter que ces produits ne sont pas classés comme médicaments et n'ont pas reçu l'approbation de la FDA pour la prévention, le traitement ou la guérison de toute condition médicale, affection ou maladie. Nous devons souligner que toute forme d'introduction corporelle de ces produits chez les humains ou les animaux est strictement interdite par la loi. Il est essentiel de respecter ces directives pour assurer la conformité aux normes légales et éthiques en matière de recherche et d'expérimentation.