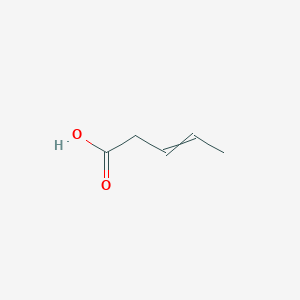
3-Pentenoic acid
Vue d'ensemble
Description
3-Pentenoic acid, also known as pent-3-enoic acid, is an organic compound with the molecular formula C(_5)H(_8)O(_2). It is a type of unsaturated carboxylic acid characterized by a double bond between the third and fourth carbon atoms in its five-carbon chain. This compound is one of the geometric isomers of pentenoic acid, which includes both cis and trans forms .
Méthodes De Préparation
Synthetic Routes and Reaction Conditions: 3-Pentenoic acid can be synthesized through the reaction of butadiene with carbon monoxide and water in the presence of a rhodium-containing catalyst and an iodide promoter. This process involves carbon monoxide stripping to separate and recover the this compound product . Another method involves using a nickel catalyst and an iodide source, where butadiene reacts with carbon monoxide in the presence of water .
Industrial Production Methods: The industrial production of this compound typically follows the aforementioned synthetic routes, utilizing either rhodium or nickel catalysts. The reaction conditions are carefully controlled to optimize yield and purity, with temperatures ranging from 60°C to 220°C and pressures between 200 psig and 4000 psig .
Analyse Des Réactions Chimiques
Types of Reactions: 3-Pentenoic acid undergoes various chemical reactions, including:
Oxidation: It can be oxidized to produce corresponding aldehydes or ketones.
Reduction: Hydrogenation of the double bond yields pentanoic acid.
Substitution: The carboxyl group can participate in esterification reactions to form esters.
Common Reagents and Conditions:
Oxidation: Common oxidizing agents include potassium permanganate and chromium trioxide.
Reduction: Catalytic hydrogenation using palladium or platinum catalysts.
Substitution: Esterification typically involves alcohols and acid catalysts like sulfuric acid.
Major Products:
Oxidation: Produces aldehydes or ketones.
Reduction: Yields pentanoic acid.
Substitution: Forms esters such as ethyl pentenoate.
Applications De Recherche Scientifique
Organic Synthesis
3-Pentenoic acid serves as a valuable intermediate in organic synthesis. It is utilized in the production of various chemical compounds, including esters and amides. The ability to undergo reactions such as esterification and amidation makes it a versatile building block in synthetic organic chemistry.
Key Reactions:
- Esterification: this compound can react with alcohols to form esters, which are important in the production of fragrances and flavorings.
- Amidation: The acid can also form amides with amines, which are crucial in pharmaceutical applications.
Pharmaceutical Applications
Recent studies have highlighted the potential of this compound and its derivatives in pharmaceutical formulations. Notably, it has been investigated for its role as a precursor in the synthesis of bioactive compounds.
Case Study:
- Migraine Treatment: Research indicates that this compound (referred to as CGP 40116) may influence synaptic excitability in migraine with aura conditions. This suggests a possible therapeutic application in managing migraine symptoms .
Material Science
In material science, this compound is explored for its potential use in polymerization processes. Its unsaturated nature allows it to participate in radical polymerization, leading to the development of new polymers with desired properties.
Applications:
- Polymer Production: The compound can be incorporated into polymer matrices, enhancing their mechanical and thermal properties.
- Coatings and Adhesives: Due to its reactivity, this compound is suitable for formulating coatings and adhesives that require specific adhesion characteristics.
Chemical Research
This compound has been studied for its reactions with various agents, providing insights into oxidative stress and cellular responses. For instance, its interaction with hypochlorous acid (HOCl) has been modeled to understand lipid double bond reactions .
Research Findings:
- The reaction kinetics of this compound with HOCl has been quantified, yielding significant data on its stability under oxidative conditions .
Environmental Applications
The compound's reactivity also opens avenues for environmental applications, particularly in the degradation of pollutants. Its derivatives can be designed to interact with environmental contaminants, facilitating their breakdown.
Potential Studies:
- Investigating the use of this compound derivatives as biodegradable surfactants or agents for pollutant remediation.
Mécanisme D'action
The mechanism of action of 3-pentenoic acid involves its interaction with molecular targets such as enzymes and receptors. The double bond in its structure allows it to participate in various biochemical reactions, potentially inhibiting or activating specific pathways. For instance, it can act as a substrate for enzymes involved in fatty acid metabolism .
Comparaison Avec Des Composés Similaires
2-Pentenoic acid: Differentiated by the position of the double bond between the second and third carbon atoms.
4-Pentenoic acid: Has the double bond between the fourth and fifth carbon atoms.
3-Butenoic acid: A shorter chain analog with a similar double bond structure.
Uniqueness: 3-Pentenoic acid is unique due to its specific double bond position, which influences its reactivity and interactions in chemical and biological systems. This positional isomerism allows for distinct applications and properties compared to its analogs .
Activité Biologique
3-Pentenoic acid, a carboxylic acid with the molecular formula , has garnered attention for its biological activities, particularly in the context of neuropharmacology and potential applications in food science. This article reviews the biological activity of this compound, focusing on its mechanisms of action, effects on neurotransmission, and its potential as a food additive.
- IUPAC Name : this compound
- Molecular Formula :
- CAS Number : 5204-64-8
- Structure :
Glutamate Antagonism
This compound acts as a competitive antagonist at glutamate receptors, particularly the NMDA receptor. This receptor is crucial for synaptic plasticity and memory function. The modulation of NMDA receptor activity is significant in various neuropsychiatric disorders, including schizophrenia. The ability of this compound to inhibit glutamate signaling suggests a potential therapeutic role in managing symptoms associated with NMDA receptor hypofunction .
Neuroprotective Effects
Research indicates that this compound may exert neuroprotective effects by stabilizing neuronal membranes and reducing excitotoxicity caused by excessive glutamate. This property is particularly relevant in conditions such as stroke and neurodegenerative diseases, where glutamate-induced neuronal damage is a concern .
Biological Activity in Food Science
This compound is also investigated for its role as a food additive due to its flavoring properties. It is classified within the group of methyl-branched fatty acids, which are known for their unique sensory attributes. The compound's presence in food products can enhance flavor profiles while potentially offering health benefits through its bioactive properties .
Antimicrobial Activity
A study evaluating various organic acids, including this compound, demonstrated significant antimicrobial activity against certain bacterial strains. The results indicated that this compound could inhibit the growth of pathogens like Escherichia coli and Staphylococcus aureus, suggesting its potential use as a natural preservative in food products .
Compound | Bacterial Strain | Inhibition Zone (mm) |
---|---|---|
This compound | E. coli | 15 |
S. aureus | 12 |
Toxicological Assessment
The Joint FAO/WHO Expert Committee has evaluated the safety of various food additives, including this compound. The committee concluded that while further studies are necessary to establish acceptable daily intakes (ADIs), initial findings suggest that it poses minimal risk when used within recommended limits .
Propriétés
Numéro CAS |
1617-32-9 |
---|---|
Formule moléculaire |
C5H8O2 |
Poids moléculaire |
100.12 g/mol |
Nom IUPAC |
pent-3-enoic acid |
InChI |
InChI=1S/C5H8O2/c1-2-3-4-5(6)7/h2-3H,4H2,1H3,(H,6,7) |
Clé InChI |
UIUWNILCHFBLEQ-UHFFFAOYSA-N |
SMILES |
CC=CCC(=O)O |
SMILES isomérique |
C/C=C/CC(=O)O |
SMILES canonique |
CC=CCC(=O)O |
Key on ui other cas no. |
5204-64-8 1617-32-9 |
Pictogrammes |
Corrosive |
Synonymes |
3-PENTENOIC ACID; RARECHEM AL BO 0169; TRANS-3-PENTENOIC ACID; (E)-3-Pentenoicacid; (E)-CH3CH=CHCH2COOH; 3-Pentenoicacid,(3E)-; 3-Pentenoicacid,(E)-; E-3-Pentenoicacid |
Origine du produit |
United States |
Retrosynthesis Analysis
AI-Powered Synthesis Planning: Our tool employs the Template_relevance Pistachio, Template_relevance Bkms_metabolic, Template_relevance Pistachio_ringbreaker, Template_relevance Reaxys, Template_relevance Reaxys_biocatalysis model, leveraging a vast database of chemical reactions to predict feasible synthetic routes.
One-Step Synthesis Focus: Specifically designed for one-step synthesis, it provides concise and direct routes for your target compounds, streamlining the synthesis process.
Accurate Predictions: Utilizing the extensive PISTACHIO, BKMS_METABOLIC, PISTACHIO_RINGBREAKER, REAXYS, REAXYS_BIOCATALYSIS database, our tool offers high-accuracy predictions, reflecting the latest in chemical research and data.
Strategy Settings
Precursor scoring | Relevance Heuristic |
---|---|
Min. plausibility | 0.01 |
Model | Template_relevance |
Template Set | Pistachio/Bkms_metabolic/Pistachio_ringbreaker/Reaxys/Reaxys_biocatalysis |
Top-N result to add to graph | 6 |
Feasible Synthetic Routes
Avertissement et informations sur les produits de recherche in vitro
Veuillez noter que tous les articles et informations sur les produits présentés sur BenchChem sont destinés uniquement à des fins informatives. Les produits disponibles à l'achat sur BenchChem sont spécifiquement conçus pour des études in vitro, qui sont réalisées en dehors des organismes vivants. Les études in vitro, dérivées du terme latin "in verre", impliquent des expériences réalisées dans des environnements de laboratoire contrôlés à l'aide de cellules ou de tissus. Il est important de noter que ces produits ne sont pas classés comme médicaments et n'ont pas reçu l'approbation de la FDA pour la prévention, le traitement ou la guérison de toute condition médicale, affection ou maladie. Nous devons souligner que toute forme d'introduction corporelle de ces produits chez les humains ou les animaux est strictement interdite par la loi. Il est essentiel de respecter ces directives pour assurer la conformité aux normes légales et éthiques en matière de recherche et d'expérimentation.