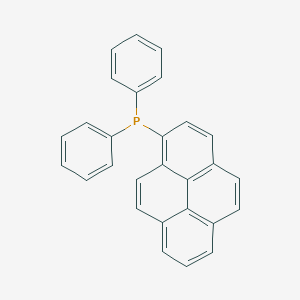
Diphényl-1-pyrènylphosphine
Vue d'ensemble
Description
Diphenyl-1-pyrenylphosphine is a unique organophosphorus compound known for its distinctive photophysical properties. It is a derivative of pyrene, a polycyclic aromatic hydrocarbon, and is characterized by the presence of a phosphorus atom bonded to a pyrene moiety and two phenyl groups. This compound exhibits remarkable third-order nonlinear optical properties and is widely used as a fluorescent probe in various scientific applications .
Applications De Recherche Scientifique
Diphenyl-1-pyrenylphosphine is extensively used in scientific research due to its unique properties:
Mécanisme D'action
Target of Action
Diphenyl-1-pyrenylphosphine (DPPP) is a fluoregenic peroxide reactive probe . It primarily targets hydroperoxides . Hydroperoxides are reactive oxygen species that play a crucial role in various biological processes, including cell signaling and inflammation .
Mode of Action
DPPP reacts stoichiometrically with hydroperoxides to yield the fluorescent molecule diphenyl-1-pyrenylphosphine oxide (DPPP-O) . This reaction is photo-triggered, meaning it is initiated by light . The interaction results in a unique aggregation-induced emission (AIE)/aggregation-caused quenching (ACQ) transition . This transition is accompanied by a remarkable third-order nonlinear optical signal change , which originates from the photo-induced oxidation of a phosphorus atom .
Biochemical Pathways
The biochemical pathways affected by DPPP are those involving lipid peroxidation . Lipid peroxidation refers to the oxidative degradation of lipids, a process in which free radicals “steal” electrons from the lipids in cell membranes, resulting in cell damage . DPPP has been used as a fluorescent probe for the detection of lipid peroxidation in living cells .
Pharmacokinetics
It’s known that dppp can be integrated into u937 cells , suggesting it has the ability to penetrate cell membranes
Result of Action
The result of DPPP’s action is the generation of a fluorescent signal . This signal change is significant and can be monitored using specific excitation and emission wavelengths . The fluorescence of DPPP-O can be monitored using excitation and emission wavelengths of 351 nm and 380 nm, respectively . This allows for the detection and quantification of hydroperoxides in the target cells .
Action Environment
The action of DPPP is influenced by environmental factors such as light and the presence of hydroperoxides . The photo-triggered reaction of DPPP requires light, and the intensity of the resulting fluorescent signal may be influenced by the intensity of the light source . Additionally, the presence and concentration of hydroperoxides in the environment will directly affect the extent of DPPP’s reaction .
Analyse Biochimique
Biochemical Properties
Diphenyl-1-pyrenylphosphine exhibits a unique photo-triggered AIE/ACQ transition with a remarkable third-order nonlinear optical signal change . This change is proved to originate from the photo-induced oxidation of a phosphorus atom
Cellular Effects
It has been integrated into U937 cells, a human leukemic monocyte lymphoma cell line . The compound’s unique properties, such as its photo-triggered AIE/ACQ transition, could potentially influence cell function, including impacts on cell signaling pathways, gene expression, and cellular metabolism .
Molecular Mechanism
The molecular mechanism of Diphenyl-1-pyrenylphosphine involves a photo-triggered AIE/ACQ transition, which is a result of the photo-induced oxidation of a phosphorus atom . This results in a remarkable third-order nonlinear optical signal change
Temporal Effects in Laboratory Settings
In laboratory settings, Diphenyl-1-pyrenylphosphine has been shown to exhibit a unique photo-triggered AIE/ACQ transition . The fluorescent product of Diphenyl-1-pyrenylphosphine, DPPP oxide, was found to be quite stable in the membrane of living cells for at least 2 days .
Metabolic Pathways
Its unique photo-triggered AIE/ACQ transition, resulting from the photo-induced oxidation of a phosphorus atom, suggests that it may interact with certain enzymes or cofactors .
Subcellular Localization
It has been integrated into U937 cells , suggesting that it can penetrate cell membranes
Méthodes De Préparation
Synthetic Routes and Reaction Conditions: Diphenyl-1-pyrenylphosphine is typically synthesized through a multi-step process involving the reaction of pyrene with chlorodiphenylphosphine. The reaction is carried out under an inert atmosphere, such as nitrogen or argon, to prevent oxidation. The reaction mixture is usually heated to facilitate the formation of the desired product .
Industrial Production Methods: In an industrial setting, the synthesis of diphenyl-1-pyrenylphosphine involves large-scale reactions with stringent control over reaction conditions to ensure high yield and purity. The process includes purification steps such as recrystallization and chromatography to isolate the compound from by-products and impurities .
Analyse Des Réactions Chimiques
Types of Reactions: Diphenyl-1-pyrenylphosphine undergoes various chemical reactions, including:
Oxidation: The compound reacts with oxidizing agents to form diphenyl-1-pyrenylphosphine oxide.
Substitution: It can participate in substitution reactions where the phenyl groups are replaced by other substituents.
Common Reagents and Conditions:
Oxidizing Agents: Hydrogen peroxide, m-chloroperbenzoic acid.
Solvents: Dichloromethane, toluene, and other non-polar solvents.
Major Products:
Diphenyl-1-pyrenylphosphine oxide: Formed through oxidation reactions.
Comparaison Avec Des Composés Similaires
Triphenylphosphine: Another organophosphorus compound with similar reactivity but different photophysical properties.
Diphenylphosphine: Lacks the pyrene moiety, resulting in different applications and properties.
Uniqueness: Diphenyl-1-pyrenylphosphine is unique due to its combination of a pyrene moiety and phosphorus atom, which imparts distinctive photophysical properties and makes it highly effective as a fluorescent probe for detecting oxidative processes .
Propriétés
IUPAC Name |
diphenyl(pyren-1-yl)phosphane | |
---|---|---|
Source | PubChem | |
URL | https://pubchem.ncbi.nlm.nih.gov | |
Description | Data deposited in or computed by PubChem | |
InChI |
InChI=1S/C28H19P/c1-3-10-23(11-4-1)29(24-12-5-2-6-13-24)26-19-17-22-15-14-20-8-7-9-21-16-18-25(26)28(22)27(20)21/h1-19H | |
Source | PubChem | |
URL | https://pubchem.ncbi.nlm.nih.gov | |
Description | Data deposited in or computed by PubChem | |
InChI Key |
DSYGKYCYNVHCNQ-UHFFFAOYSA-N | |
Source | PubChem | |
URL | https://pubchem.ncbi.nlm.nih.gov | |
Description | Data deposited in or computed by PubChem | |
Canonical SMILES |
C1=CC=C(C=C1)P(C2=CC=CC=C2)C3=C4C=CC5=CC=CC6=C5C4=C(C=C6)C=C3 | |
Source | PubChem | |
URL | https://pubchem.ncbi.nlm.nih.gov | |
Description | Data deposited in or computed by PubChem | |
Molecular Formula |
C28H19P | |
Source | PubChem | |
URL | https://pubchem.ncbi.nlm.nih.gov | |
Description | Data deposited in or computed by PubChem | |
DSSTOX Substance ID |
DTXSID60149186 | |
Record name | Diphenyl-1-pyrenylphosphine | |
Source | EPA DSSTox | |
URL | https://comptox.epa.gov/dashboard/DTXSID60149186 | |
Description | DSSTox provides a high quality public chemistry resource for supporting improved predictive toxicology. | |
Molecular Weight |
386.4 g/mol | |
Source | PubChem | |
URL | https://pubchem.ncbi.nlm.nih.gov | |
Description | Data deposited in or computed by PubChem | |
CAS No. |
110231-30-6 | |
Record name | Diphenyl-1-pyrenylphosphine | |
Source | ChemIDplus | |
URL | https://pubchem.ncbi.nlm.nih.gov/substance/?source=chemidplus&sourceid=0110231306 | |
Description | ChemIDplus is a free, web search system that provides access to the structure and nomenclature authority files used for the identification of chemical substances cited in National Library of Medicine (NLM) databases, including the TOXNET system. | |
Record name | Diphenyl-1-pyrenylphosphine | |
Source | EPA DSSTox | |
URL | https://comptox.epa.gov/dashboard/DTXSID60149186 | |
Description | DSSTox provides a high quality public chemistry resource for supporting improved predictive toxicology. | |
Record name | Diphenyl-1-pyrenylphosphine (This product is only available for selling domestically in Japan) | |
Source | European Chemicals Agency (ECHA) | |
URL | https://echa.europa.eu/information-on-chemicals | |
Description | The European Chemicals Agency (ECHA) is an agency of the European Union which is the driving force among regulatory authorities in implementing the EU's groundbreaking chemicals legislation for the benefit of human health and the environment as well as for innovation and competitiveness. | |
Explanation | Use of the information, documents and data from the ECHA website is subject to the terms and conditions of this Legal Notice, and subject to other binding limitations provided for under applicable law, the information, documents and data made available on the ECHA website may be reproduced, distributed and/or used, totally or in part, for non-commercial purposes provided that ECHA is acknowledged as the source: "Source: European Chemicals Agency, http://echa.europa.eu/". Such acknowledgement must be included in each copy of the material. ECHA permits and encourages organisations and individuals to create links to the ECHA website under the following cumulative conditions: Links can only be made to webpages that provide a link to the Legal Notice page. | |
Synthesis routes and methods
Procedure details
Retrosynthesis Analysis
AI-Powered Synthesis Planning: Our tool employs the Template_relevance Pistachio, Template_relevance Bkms_metabolic, Template_relevance Pistachio_ringbreaker, Template_relevance Reaxys, Template_relevance Reaxys_biocatalysis model, leveraging a vast database of chemical reactions to predict feasible synthetic routes.
One-Step Synthesis Focus: Specifically designed for one-step synthesis, it provides concise and direct routes for your target compounds, streamlining the synthesis process.
Accurate Predictions: Utilizing the extensive PISTACHIO, BKMS_METABOLIC, PISTACHIO_RINGBREAKER, REAXYS, REAXYS_BIOCATALYSIS database, our tool offers high-accuracy predictions, reflecting the latest in chemical research and data.
Strategy Settings
Precursor scoring | Relevance Heuristic |
---|---|
Min. plausibility | 0.01 |
Model | Template_relevance |
Template Set | Pistachio/Bkms_metabolic/Pistachio_ringbreaker/Reaxys/Reaxys_biocatalysis |
Top-N result to add to graph | 6 |
Feasible Synthetic Routes
Avertissement et informations sur les produits de recherche in vitro
Veuillez noter que tous les articles et informations sur les produits présentés sur BenchChem sont destinés uniquement à des fins informatives. Les produits disponibles à l'achat sur BenchChem sont spécifiquement conçus pour des études in vitro, qui sont réalisées en dehors des organismes vivants. Les études in vitro, dérivées du terme latin "in verre", impliquent des expériences réalisées dans des environnements de laboratoire contrôlés à l'aide de cellules ou de tissus. Il est important de noter que ces produits ne sont pas classés comme médicaments et n'ont pas reçu l'approbation de la FDA pour la prévention, le traitement ou la guérison de toute condition médicale, affection ou maladie. Nous devons souligner que toute forme d'introduction corporelle de ces produits chez les humains ou les animaux est strictement interdite par la loi. Il est essentiel de respecter ces directives pour assurer la conformité aux normes légales et éthiques en matière de recherche et d'expérimentation.