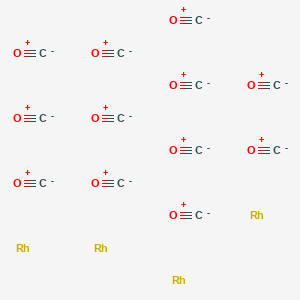
Tetrarhodium dodecacarbonyl
- Cliquez sur DEMANDE RAPIDE pour recevoir un devis de notre équipe d'experts.
- Avec des produits de qualité à un prix COMPÉTITIF, vous pouvez vous concentrer davantage sur votre recherche.
Vue d'ensemble
Description
Tetrarhodium dodecacarbonyl is a chemical compound with the formula Rh₄(CO)₁₂. This dark-red crystalline solid is the smallest binary rhodium carbonyl that can be handled as a solid under ambient conditions. It is primarily used as a catalyst in organic synthesis .
Applications De Recherche Scientifique
Tetrarhodium dodecacarbonyl is widely used in scientific research due to its catalytic properties. It is used as a catalyst in organic synthesis, particularly in hydroformylation reactions .
Mécanisme D'action
Target of Action
Tetrarhodium dodecacarbonyl, with the chemical formula Rh4(CO)12 , is primarily used as a catalyst in organic synthesis . Its primary targets are the reactants in the organic reactions it catalyzes.
Mode of Action
The compound features a tetrahedral array of four Rh atoms with nine terminal CO ligands and three bridging CO ligands . This structure allows it to interact with its targets and facilitate various organic reactions.
Action Environment
The action, efficacy, and stability of this compound can be influenced by various environmental factors. For instance, it is known to be sensitive to air and light, and it should be stored at temperatures between 2-8°C . Furthermore, its solubility in chlorocarbons, toluene, and tetrahydrofuran suggests that the solvent used in the reaction could also influence its action and stability.
Méthodes De Préparation
Synthetic Routes and Reaction Conditions
Tetrarhodium dodecacarbonyl can be synthesized by treating an aqueous solution of rhodium trichloride with activated copper metal under an atmosphere of carbon monoxide. The reaction is as follows :
[ 4 \text{RhCl}_3(\text{H}_2\text{O})_3 + 8 \text{Cu} + 22 \text{CO} \rightarrow \text{Rh}4(\text{CO}){12} + 2 \text{CO}_2 + 8 \text{Cu}(\text{CO})\text{Cl} + 4 \text{HCl} + 10 \text{H}_2\text{O} ]
Alternatively, it can be prepared by treating a methanolic solution of rhodium trichloride with carbon monoxide to afford H[RhCl₂(CO)₂], followed by carbonylation in the presence of sodium citrate .
Industrial Production Methods
The industrial production methods for this compound are similar to the laboratory synthesis methods, involving the reaction of rhodium trichloride with carbon monoxide in the presence of a reducing agent such as activated copper .
Analyse Des Réactions Chimiques
Types of Reactions
Tetrarhodium dodecacarbonyl undergoes various types of reactions, including thermal substitution and decomposition .
-
Thermal Substitution: : The compound undergoes thermal substitution with phosphine ligands (L) as follows: [ \text{Rh}4(\text{CO}){12} + n \text{L} \rightarrow \text{Rh}4(\text{CO}){12-n}\text{L}_n + n \text{CO} ]
-
Decomposition: : this compound quantitatively decomposes in boiling hexane to afford hexadecacarbonylhexarhodium: [ 3 \text{Rh}4(\text{CO}){12} \rightarrow 2 \text{Rh}6(\text{CO}){16} + 4 \text{CO} ]
Common Reagents and Conditions
Common reagents used in reactions with this compound include phosphine ligands and solvents such as hexane. The reactions typically occur under thermal conditions .
Major Products
The major products formed from the reactions of this compound include substituted rhodium carbonyl complexes and hexadecacarbonylhexarhodium .
Comparaison Avec Des Composés Similaires
Similar Compounds
- Tetracobalt dodecacarbonyl
- Tetrairidium dodecacarbonyl
- Hexadecacarbonylhexarhodium
- Rhodium (III) chloride
Uniqueness
Tetrarhodium dodecacarbonyl is unique due to its stability as the smallest binary rhodium carbonyl that can be handled as a solid under ambient conditions. Its catalytic properties and ability to undergo thermal substitution and decomposition reactions make it distinct from other similar compounds .
Propriétés
IUPAC Name |
carbon monoxide;rhodium |
Source
|
---|---|---|
Source | PubChem | |
URL | https://pubchem.ncbi.nlm.nih.gov | |
Description | Data deposited in or computed by PubChem | |
InChI |
InChI=1S/12CO.4Rh/c12*1-2;;;; |
Source
|
Source | PubChem | |
URL | https://pubchem.ncbi.nlm.nih.gov | |
Description | Data deposited in or computed by PubChem | |
InChI Key |
LVGLLYVYRZMJIN-UHFFFAOYSA-N |
Source
|
Source | PubChem | |
URL | https://pubchem.ncbi.nlm.nih.gov | |
Description | Data deposited in or computed by PubChem | |
Canonical SMILES |
[C-]#[O+].[C-]#[O+].[C-]#[O+].[C-]#[O+].[C-]#[O+].[C-]#[O+].[C-]#[O+].[C-]#[O+].[C-]#[O+].[C-]#[O+].[C-]#[O+].[C-]#[O+].[Rh].[Rh].[Rh].[Rh] |
Source
|
Source | PubChem | |
URL | https://pubchem.ncbi.nlm.nih.gov | |
Description | Data deposited in or computed by PubChem | |
Molecular Formula |
C12O12Rh4 |
Source
|
Source | PubChem | |
URL | https://pubchem.ncbi.nlm.nih.gov | |
Description | Data deposited in or computed by PubChem | |
Molecular Weight |
747.74 g/mol |
Source
|
Source | PubChem | |
URL | https://pubchem.ncbi.nlm.nih.gov | |
Description | Data deposited in or computed by PubChem | |
CAS No. |
19584-30-6 |
Source
|
Record name | Tri-μ-carbonylnonacarbonyl-tetrahedro-tetrarhodium | |
Source | European Chemicals Agency (ECHA) | |
URL | https://echa.europa.eu/substance-information/-/substanceinfo/100.039.232 | |
Description | The European Chemicals Agency (ECHA) is an agency of the European Union which is the driving force among regulatory authorities in implementing the EU's groundbreaking chemicals legislation for the benefit of human health and the environment as well as for innovation and competitiveness. | |
Explanation | Use of the information, documents and data from the ECHA website is subject to the terms and conditions of this Legal Notice, and subject to other binding limitations provided for under applicable law, the information, documents and data made available on the ECHA website may be reproduced, distributed and/or used, totally or in part, for non-commercial purposes provided that ECHA is acknowledged as the source: "Source: European Chemicals Agency, http://echa.europa.eu/". Such acknowledgement must be included in each copy of the material. ECHA permits and encourages organisations and individuals to create links to the ECHA website under the following cumulative conditions: Links can only be made to webpages that provide a link to the Legal Notice page. | |
Q1: What is the molecular formula, weight, and spectroscopic data for tetrarhodium dodecacarbonyl?
A1: this compound has the molecular formula Rh4(CO)12 and a molecular weight of 605.58 g/mol. Its infrared spectrum is characterized by strong absorptions in the carbonyl stretching region (νCO), typically observed between 2036-2121 cm-1. []
Q2: What are the main catalytic properties of this compound?
A2: this compound is a versatile homogeneous catalyst known for its activity in various reactions, including hydroformylation, silylformylation, and the addition of aromatic heterocyclic compounds to acetylenes. [, , ]
Q3: How does this compound interact with alkynes in a hydroformylation reaction?
A3: Research using in-situ high-pressure infrared spectroscopy revealed that both monosubstituted and disubstituted alkynes react quantitatively with Rh4(CO)12 under CO and CO/H2 mixtures. [] This interaction ultimately leads to the formation of various alkyne-rhodium complexes, the nature of which depends on the alkyne's substituents.
Q4: What is the reaction mechanism of alkene hydroformylation catalyzed by this compound?
A4: While the exact mechanism depends on the specific alkene, studies using in-situ infrared spectroscopy have identified acyl rhodium tetracarbonyl intermediates during the hydroformylation of various alkenes. [] This suggests a mechanism involving alkene coordination, CO insertion, hydrogenolysis, and product elimination.
Q5: What is the role of this compound in the reduction of nitric oxide?
A5: Studies have shown that this compound, in an aqueous alkaline solution, can catalyze the reduction of nitric oxide to ammonia. [] Interestingly, this reaction also incorporates the water gas shift reaction, highlighting the complex interplay of reactants and intermediates in this catalytic system.
Q6: Can you provide an example of this compound's activity towards aromatic heterocyclic compounds?
A6: Rh4(CO)12 catalyzes the addition of furan, thiophene, and N-methylpyrrole to diphenylacetylene, yielding vinyl-substituted aromatic heterocyclic compounds. [] This reaction demonstrates the catalyst's ability to activate C-H bonds in heterocycles and promote carbon-carbon bond formation.
Q7: How does the structure of this compound relate to its catalytic activity?
A7: The tetrahedral arrangement of rhodium atoms in Rh4(CO)12, along with the labile carbonyl ligands, allows for the creation of coordinatively unsaturated species. These species can readily interact with substrates, facilitating bond activation and subsequent catalytic transformations. []
Q8: What are the limitations of this compound as a catalyst?
A8: One significant limitation is its sensitivity to alkynes. Even trace amounts can poison the catalyst, hindering its activity in hydroformylation reactions. [] Additionally, like many homogeneous catalysts, its separation and recovery from the reaction mixture can be challenging.
Avertissement et informations sur les produits de recherche in vitro
Veuillez noter que tous les articles et informations sur les produits présentés sur BenchChem sont destinés uniquement à des fins informatives. Les produits disponibles à l'achat sur BenchChem sont spécifiquement conçus pour des études in vitro, qui sont réalisées en dehors des organismes vivants. Les études in vitro, dérivées du terme latin "in verre", impliquent des expériences réalisées dans des environnements de laboratoire contrôlés à l'aide de cellules ou de tissus. Il est important de noter que ces produits ne sont pas classés comme médicaments et n'ont pas reçu l'approbation de la FDA pour la prévention, le traitement ou la guérison de toute condition médicale, affection ou maladie. Nous devons souligner que toute forme d'introduction corporelle de ces produits chez les humains ou les animaux est strictement interdite par la loi. Il est essentiel de respecter ces directives pour assurer la conformité aux normes légales et éthiques en matière de recherche et d'expérimentation.