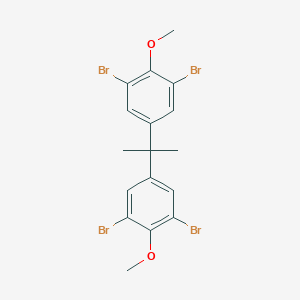
Tetrabromobisphenol A bismethyl ether
Vue d'ensemble
Description
Molecular Structure Analysis
The molecular structure of TBBPA-BAE is represented by the formula C17H16Br4O2 . More detailed structural analysis is not available in the retrieved papers.Physical And Chemical Properties Analysis
TBBPA-BAE is a white to off-white crystalline solid with a slight odor . Its relative molecular mass is 943.9; the melting point is 90° to 100°C (95°C); and the specific gravity is 0.7 to 0.9 g/cm 3 .Applications De Recherche Scientifique
Flame Retardancy in Consumer Products
Tetrabromobisphenol A dimethyl ether: is primarily employed as a flame retardant in various consumer products. Its high bromine content makes it effective in reducing the flammability of products such as furniture, circuit boards, textiles, polystyrene foams, epoxy resins, and padding materials . The compound helps in meeting stringent safety standards and is crucial in preventing fire-related accidents.
Environmental Fate and Biodegradation
Research has shown that Tetrabromobisphenol A dimethyl ether can undergo biotransformation in environmental systems. For instance, studies involving pumpkin plants have demonstrated the compound’s ability to be metabolized back to TBBPA, indicating a dynamic environmental fate and potential for biodegradation . This is significant for understanding the environmental impact and developing strategies for remediation.
Human Biomonitoring and Toxicity Analysis
As an endocrine disruptor, Tetrabromobisphenol A dimethyl ether has been the subject of human biomonitoring studies to assess its presence in human samples and its toxic effects. These studies are crucial for evaluating the risks associated with exposure and for establishing safety guidelines .
Analytical Chemistry and Detection Methods
The compound’s detection and quantification in various matrices are essential for environmental monitoring and safety assessments. Advanced analytical techniques, including chromatography and mass spectrometry, are utilized to detect and measure the levels of Tetrabromobisphenol A dimethyl ether in different environments .
Neurotoxicity Research
There is ongoing research to determine the neurotoxic effects of Tetrabromobisphenol A dimethyl ether . Studies involving model organisms like zebrafish are conducted to observe any behavioral changes and assess the neurotoxic potential of the compound .
Treatment and Removal from the Environment
Innovative treatment methods are being explored to remove Tetrabromobisphenol A dimethyl ether from the environment. This includes adsorbent-based treatments and advanced oxidation processes to degrade the compound and reduce its environmental footprint .
Endocrine Disruption and Health Implications
Finally, the role of Tetrabromobisphenol A dimethyl ether as an endocrine disruptor is a significant area of research. Studies aim to understand its mode of action, potential health implications, and ways to mitigate its effects on human health and the environment .
Mécanisme D'action
Target of Action
Tetrabromobisphenol A dimethyl ether (TBBPA DME), also known as Tetrabromobisphenol A bismethyl ether or 1,3-dibromo-5-[2-(3,5-dibromo-4-methoxyphenyl)propan-2-yl]-2-methoxybenzene, is a derivative of Tetrabromobisphenol A (TBBPA). TBBPA is a known endocrine disruptor . The primary targets of TBBPA DME are likely to be similar to those of TBBPA, which include both estrogens and androgens . It may also interfere with the thyroid hormone thyroxin (T4) by binding more strongly to the transport protein transthyretin than T4 does .
Mode of Action
It is known that tbbpa dme can be biotransformed back to tbbpa in certain organisms, such as pumpkin plants . This suggests that TBBPA DME might interact with its targets in a similar way to TBBPA. As an endocrine disruptor, TBBPA may interfere with hormone signaling pathways, leading to changes in cellular function .
Biochemical Pathways
TBBPA DME likely affects the same biochemical pathways as TBBPA. As an endocrine disruptor, TBBPA can interfere with the normal functioning of the endocrine system, which includes a wide range of hormonal signaling pathways .
Pharmacokinetics
It is known that tbbpa and its derivatives can be absorbed and accumulate in various aquatic organisms . The rate of metabolism of these compounds is relatively slow
Result of Action
The molecular and cellular effects of TBBPA DME’s action are likely to be similar to those of TBBPA. As an endocrine disruptor, TBBPA can cause a variety of effects, including neurobehavioral and immunotoxic effects, oxidative stress, and apoptosis . If TBBPA DME acts in a similar way, it may have similar effects.
Action Environment
The action of TBBPA DME can be influenced by various environmental factors. For example, the pH, temperature, and natural organic matter concentration of water can affect the abiotic methylation of TBBPA . Additionally, TBBPA DME has been found to accumulate more in the roots of pumpkin plants and translocate up to stems and leaves compared with TBBPA . This suggests that the plant environment can influence the action and efficacy of TBBPA DME.
Safety and Hazards
Orientations Futures
Studies on exposure routes in humans, a combination of detection methods, adsorbent-based treatments and degradation of TBBPA are in the preliminary phase and have several limitations . Therefore, in-depth studies on these subjects should be considered to enhance the accurate body load of non-invasive matrix, external exposure levels, optimal design of combined detection techniques, and degrading technology of TBBPA .
Propriétés
IUPAC Name |
1,3-dibromo-5-[2-(3,5-dibromo-4-methoxyphenyl)propan-2-yl]-2-methoxybenzene | |
---|---|---|
Source | PubChem | |
URL | https://pubchem.ncbi.nlm.nih.gov | |
Description | Data deposited in or computed by PubChem | |
InChI |
InChI=1S/C17H16Br4O2/c1-17(2,9-5-11(18)15(22-3)12(19)6-9)10-7-13(20)16(23-4)14(21)8-10/h5-8H,1-4H3 | |
Source | PubChem | |
URL | https://pubchem.ncbi.nlm.nih.gov | |
Description | Data deposited in or computed by PubChem | |
InChI Key |
XRQKNNNAKHZPSP-UHFFFAOYSA-N | |
Source | PubChem | |
URL | https://pubchem.ncbi.nlm.nih.gov | |
Description | Data deposited in or computed by PubChem | |
Canonical SMILES |
CC(C)(C1=CC(=C(C(=C1)Br)OC)Br)C2=CC(=C(C(=C2)Br)OC)Br | |
Source | PubChem | |
URL | https://pubchem.ncbi.nlm.nih.gov | |
Description | Data deposited in or computed by PubChem | |
Molecular Formula |
C17H16Br4O2 | |
Source | PubChem | |
URL | https://pubchem.ncbi.nlm.nih.gov | |
Description | Data deposited in or computed by PubChem | |
DSSTOX Substance ID |
DTXSID10865889 | |
Record name | Tetrabromobisphenol A dimethyl ether | |
Source | EPA DSSTox | |
URL | https://comptox.epa.gov/dashboard/DTXSID10865889 | |
Description | DSSTox provides a high quality public chemistry resource for supporting improved predictive toxicology. | |
Molecular Weight |
571.9 g/mol | |
Source | PubChem | |
URL | https://pubchem.ncbi.nlm.nih.gov | |
Description | Data deposited in or computed by PubChem | |
Product Name |
Tetrabromobisphenol A bismethyl ether | |
CAS RN |
37853-61-5 | |
Record name | Tetrabromobisphenol A dimethyl ether | |
Source | CAS Common Chemistry | |
URL | https://commonchemistry.cas.org/detail?cas_rn=37853-61-5 | |
Description | CAS Common Chemistry is an open community resource for accessing chemical information. Nearly 500,000 chemical substances from CAS REGISTRY cover areas of community interest, including common and frequently regulated chemicals, and those relevant to high school and undergraduate chemistry classes. This chemical information, curated by our expert scientists, is provided in alignment with our mission as a division of the American Chemical Society. | |
Explanation | The data from CAS Common Chemistry is provided under a CC-BY-NC 4.0 license, unless otherwise stated. | |
Record name | Benzene, 1,1'-(1-methylethylidene)bis(3,5-dibromo-4-methoxy- | |
Source | ChemIDplus | |
URL | https://pubchem.ncbi.nlm.nih.gov/substance/?source=chemidplus&sourceid=0037853615 | |
Description | ChemIDplus is a free, web search system that provides access to the structure and nomenclature authority files used for the identification of chemical substances cited in National Library of Medicine (NLM) databases, including the TOXNET system. | |
Record name | Benzene, 1,1'-(1-methylethylidene)bis[3,5-dibromo-4-methoxy- | |
Source | EPA Chemicals under the TSCA | |
URL | https://www.epa.gov/chemicals-under-tsca | |
Description | EPA Chemicals under the Toxic Substances Control Act (TSCA) collection contains information on chemicals and their regulations under TSCA, including non-confidential content from the TSCA Chemical Substance Inventory and Chemical Data Reporting. | |
Record name | Tetrabromobisphenol A dimethyl ether | |
Source | EPA DSSTox | |
URL | https://comptox.epa.gov/dashboard/DTXSID10865889 | |
Description | DSSTox provides a high quality public chemistry resource for supporting improved predictive toxicology. | |
Record name | 4,4'-(isopropylidene)bis[2,6-dibromoanisole] m | |
Source | European Chemicals Agency (ECHA) | |
URL | https://echa.europa.eu/substance-information/-/substanceinfo/100.048.795 | |
Description | The European Chemicals Agency (ECHA) is an agency of the European Union which is the driving force among regulatory authorities in implementing the EU's groundbreaking chemicals legislation for the benefit of human health and the environment as well as for innovation and competitiveness. | |
Explanation | Use of the information, documents and data from the ECHA website is subject to the terms and conditions of this Legal Notice, and subject to other binding limitations provided for under applicable law, the information, documents and data made available on the ECHA website may be reproduced, distributed and/or used, totally or in part, for non-commercial purposes provided that ECHA is acknowledged as the source: "Source: European Chemicals Agency, http://echa.europa.eu/". Such acknowledgement must be included in each copy of the material. ECHA permits and encourages organisations and individuals to create links to the ECHA website under the following cumulative conditions: Links can only be made to webpages that provide a link to the Legal Notice page. | |
Retrosynthesis Analysis
AI-Powered Synthesis Planning: Our tool employs the Template_relevance Pistachio, Template_relevance Bkms_metabolic, Template_relevance Pistachio_ringbreaker, Template_relevance Reaxys, Template_relevance Reaxys_biocatalysis model, leveraging a vast database of chemical reactions to predict feasible synthetic routes.
One-Step Synthesis Focus: Specifically designed for one-step synthesis, it provides concise and direct routes for your target compounds, streamlining the synthesis process.
Accurate Predictions: Utilizing the extensive PISTACHIO, BKMS_METABOLIC, PISTACHIO_RINGBREAKER, REAXYS, REAXYS_BIOCATALYSIS database, our tool offers high-accuracy predictions, reflecting the latest in chemical research and data.
Strategy Settings
Precursor scoring | Relevance Heuristic |
---|---|
Min. plausibility | 0.01 |
Model | Template_relevance |
Template Set | Pistachio/Bkms_metabolic/Pistachio_ringbreaker/Reaxys/Reaxys_biocatalysis |
Top-N result to add to graph | 6 |
Feasible Synthetic Routes
Q & A
Q1: What is the environmental fate of TBBPA-DME and can it be transformed back to the more toxic Tetrabromobisphenol A (TBBPA)?
A1: While TBBPA-DME is considered a derivative of TBBPA with potentially lower toxicity, research indicates it can be biotransformed back into TBBPA. One study demonstrated that whole pumpkin plants exposed to TBBPA-DME were able to convert it back to TBBPA. [] This finding has significant implications for environmental risk assessments as it suggests TBBPA-DME may not be a safe alternative to TBBPA and could contribute to the persistence of TBBPA in the environment. [, ]
Q2: What are the potential developmental effects of exposure to TBBPA-DME?
A2: Research using zebrafish embryos has shown that exposure to TBBPA-DME can disrupt normal development. [] This disruption was linked to changes in the expression of matrix metalloproteinases, enzymes involved in the breakdown and remodeling of extracellular matrix, which play crucial roles in developmental processes. These findings raise concerns about the potential developmental toxicity of TBBPA-DME and highlight the need for further investigation into its long-term effects.
Q3: How prevalent is TBBPA-DME in the environment?
A3: Studies have detected TBBPA-DME, alongside TBBPA and its mono-methyl derivative, in various environmental samples. These include fish, sediment, and suspended particulate matter collected from European freshwater and estuarine systems. [] This detection highlights the widespread distribution of TBBPA and its derivatives in aquatic environments, raising concerns about potential ecological and human health risks. Further research is needed to understand the full extent of TBBPA-DME contamination and its potential consequences.
Avertissement et informations sur les produits de recherche in vitro
Veuillez noter que tous les articles et informations sur les produits présentés sur BenchChem sont destinés uniquement à des fins informatives. Les produits disponibles à l'achat sur BenchChem sont spécifiquement conçus pour des études in vitro, qui sont réalisées en dehors des organismes vivants. Les études in vitro, dérivées du terme latin "in verre", impliquent des expériences réalisées dans des environnements de laboratoire contrôlés à l'aide de cellules ou de tissus. Il est important de noter que ces produits ne sont pas classés comme médicaments et n'ont pas reçu l'approbation de la FDA pour la prévention, le traitement ou la guérison de toute condition médicale, affection ou maladie. Nous devons souligner que toute forme d'introduction corporelle de ces produits chez les humains ou les animaux est strictement interdite par la loi. Il est essentiel de respecter ces directives pour assurer la conformité aux normes légales et éthiques en matière de recherche et d'expérimentation.