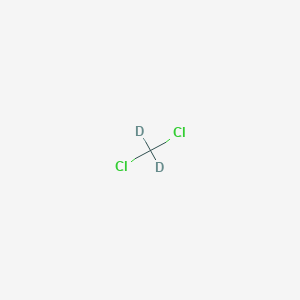
Dichloromethane-d2
Vue d'ensemble
Description
Dichloromethane-d2 is a deuterated derivative of dichloromethane . It is used as a solvent during the NMR spectral analysis of polymers . It has also been employed as a deuterated solvent for sample preparation in NMR analysis .
Synthesis Analysis
Dichloromethane-d2 can be synthesized by treating methane or chloromethane with chlorine gas at a temperature range of 400–500 °C .Molecular Structure Analysis
The molecular structure of Dichloromethane-d2 is represented by the linear formula CD2Cl2 . The molecular weight is 86.94 .Chemical Reactions Analysis
Dichloromethane-d2 is primarily used as a solvent in various chemical reactions . It is particularly useful in NMR-based research and analyses .Physical And Chemical Properties Analysis
Dichloromethane-d2 is a liquid with a refractive index of n20/D 1.422 (lit.) . It has a boiling point of 40 °C (lit.) and a density of 1.362 g/mL at 25 °C (lit.) . It is not miscible with water .Applications De Recherche Scientifique
Nuclear Magnetic Resonance (NMR) Spectroscopy
Dichloromethane-d2 is extensively used as a solvent in NMR spectroscopy. Its deuterated nature means it has a minimal NMR-active hydrogen signal, which reduces background noise and enhances the clarity of the spectral data. This makes it ideal for analyzing the structure and dynamics of organic molecules .
Mass Spectrometry
In mass spectrometry, Dichloromethane-d2 can be used to facilitate the analysis of complex mixtures by providing a clear mass shift of M+2. This shift helps in distinguishing between different isotopic species and enhances the accuracy of mass determination .
Polymer Research
The solvent properties of Dichloromethane-d2 are utilized in the study of polymers. It is particularly useful in the NMR spectral analysis of polymers, allowing for the elucidation of their structure and composition .
Pharmaceutical Research
Dichloromethane-d2 is employed in pharmaceutical research, particularly in the structural elucidation of new drugs and metabolites. Its use in NMR spectroscopy provides valuable insights into the molecular structure and interactions of pharmaceutical compounds .
Chemical Synthesis
In synthetic chemistry, Dichloromethane-d2 is used as a reaction medium, especially in reactions where deuterium incorporation is desired. It serves as a source of deuterium atoms that can be transferred to the target molecules, thus aiding in the synthesis of labeled compounds .
Analytical Chemistry
Analytical chemists use Dichloromethane-d2 for sample preparation in various analytical techniques. Its low boiling point and non-reactive nature make it an excellent solvent for preparing samples for analysis, ensuring that the integrity of the sample is maintained .
Mécanisme D'action
Target of Action
Dichloromethane-d2, also known as Dideuteromethylenechloride or Methylene chloride-d2 , is a deuterated derivative of dichloromethane . It is primarily used as a solvent during the NMR spectral analysis of polymers . The primary targets of Dichloromethane-d2 are the molecules that are being analyzed in the NMR process .
Mode of Action
Dichloromethane-d2 interacts with its targets by incorporating into drug molecules, largely as tracers for quantitation during the drug development process . Deuteration has gained attention because of its potential to affect the pharmacokinetic and metabolic profiles of drugs .
Biochemical Pathways
It is known that aerobic methylotrophic bacteria can degrade dichloromethane . The enzymological and genetic aspects of dichloromethane dehalogenation, including probable pathways used by cells to overcome accompanying stresses (acid, osmotic, and oxidative), are currently being studied .
Pharmacokinetics
The pharmacokinetics of Dichloromethane-d2 are influenced by its deuterated nature. Deuteration has been shown to affect the pharmacokinetic and metabolic profiles of drugs . .
Result of Action
The molecular and cellular effects of Dichloromethane-d2’s action are largely dependent on its role as a solvent in NMR spectral analysis . It allows for the analysis of the three-dimensional structure of molecules . .
Safety and Hazards
Orientations Futures
Propriétés
IUPAC Name |
dichloro(dideuterio)methane | |
---|---|---|
Source | PubChem | |
URL | https://pubchem.ncbi.nlm.nih.gov | |
Description | Data deposited in or computed by PubChem | |
InChI |
InChI=1S/CH2Cl2/c2-1-3/h1H2/i1D2 | |
Source | PubChem | |
URL | https://pubchem.ncbi.nlm.nih.gov | |
Description | Data deposited in or computed by PubChem | |
InChI Key |
YMWUJEATGCHHMB-DICFDUPASA-N | |
Source | PubChem | |
URL | https://pubchem.ncbi.nlm.nih.gov | |
Description | Data deposited in or computed by PubChem | |
Canonical SMILES |
C(Cl)Cl | |
Source | PubChem | |
URL | https://pubchem.ncbi.nlm.nih.gov | |
Description | Data deposited in or computed by PubChem | |
Isomeric SMILES |
[2H]C([2H])(Cl)Cl | |
Source | PubChem | |
URL | https://pubchem.ncbi.nlm.nih.gov | |
Description | Data deposited in or computed by PubChem | |
Molecular Formula |
CH2Cl2 | |
Source | PubChem | |
URL | https://pubchem.ncbi.nlm.nih.gov | |
Description | Data deposited in or computed by PubChem | |
DSSTOX Substance ID |
DTXSID80937204 | |
Record name | Dichlorodideuteriomethane | |
Source | EPA DSSTox | |
URL | https://comptox.epa.gov/dashboard/DTXSID80937204 | |
Description | DSSTox provides a high quality public chemistry resource for supporting improved predictive toxicology. | |
Molecular Weight |
86.94 g/mol | |
Source | PubChem | |
URL | https://pubchem.ncbi.nlm.nih.gov | |
Description | Data deposited in or computed by PubChem | |
Physical Description |
Colorless liquid with a penetrating odor like ether; Hygroscopic; [NTP] | |
Record name | Methylene chloride-d2 | |
Source | Haz-Map, Information on Hazardous Chemicals and Occupational Diseases | |
URL | https://haz-map.com/Agents/13654 | |
Description | Haz-Map® is an occupational health database designed for health and safety professionals and for consumers seeking information about the adverse effects of workplace exposures to chemical and biological agents. | |
Explanation | Copyright (c) 2022 Haz-Map(R). All rights reserved. Unless otherwise indicated, all materials from Haz-Map are copyrighted by Haz-Map(R). No part of these materials, either text or image may be used for any purpose other than for personal use. Therefore, reproduction, modification, storage in a retrieval system or retransmission, in any form or by any means, electronic, mechanical or otherwise, for reasons other than personal use, is strictly prohibited without prior written permission. | |
Vapor Pressure |
353.1 [mmHg] | |
Record name | Methylene chloride-d2 | |
Source | Haz-Map, Information on Hazardous Chemicals and Occupational Diseases | |
URL | https://haz-map.com/Agents/13654 | |
Description | Haz-Map® is an occupational health database designed for health and safety professionals and for consumers seeking information about the adverse effects of workplace exposures to chemical and biological agents. | |
Explanation | Copyright (c) 2022 Haz-Map(R). All rights reserved. Unless otherwise indicated, all materials from Haz-Map are copyrighted by Haz-Map(R). No part of these materials, either text or image may be used for any purpose other than for personal use. Therefore, reproduction, modification, storage in a retrieval system or retransmission, in any form or by any means, electronic, mechanical or otherwise, for reasons other than personal use, is strictly prohibited without prior written permission. | |
Product Name |
Dichloromethane-d2 | |
CAS RN |
1665-00-5 | |
Record name | Dichloromethane-d2 | |
Source | CAS Common Chemistry | |
URL | https://commonchemistry.cas.org/detail?cas_rn=1665-00-5 | |
Description | CAS Common Chemistry is an open community resource for accessing chemical information. Nearly 500,000 chemical substances from CAS REGISTRY cover areas of community interest, including common and frequently regulated chemicals, and those relevant to high school and undergraduate chemistry classes. This chemical information, curated by our expert scientists, is provided in alignment with our mission as a division of the American Chemical Society. | |
Explanation | The data from CAS Common Chemistry is provided under a CC-BY-NC 4.0 license, unless otherwise stated. | |
Record name | Dichloro(2H2)methane | |
Source | ChemIDplus | |
URL | https://pubchem.ncbi.nlm.nih.gov/substance/?source=chemidplus&sourceid=0001665005 | |
Description | ChemIDplus is a free, web search system that provides access to the structure and nomenclature authority files used for the identification of chemical substances cited in National Library of Medicine (NLM) databases, including the TOXNET system. | |
Record name | Dichlorodideuteriomethane | |
Source | EPA DSSTox | |
URL | https://comptox.epa.gov/dashboard/DTXSID80937204 | |
Description | DSSTox provides a high quality public chemistry resource for supporting improved predictive toxicology. | |
Record name | Dichloro(2H2)methane | |
Source | European Chemicals Agency (ECHA) | |
URL | https://echa.europa.eu/substance-information/-/substanceinfo/100.015.252 | |
Description | The European Chemicals Agency (ECHA) is an agency of the European Union which is the driving force among regulatory authorities in implementing the EU's groundbreaking chemicals legislation for the benefit of human health and the environment as well as for innovation and competitiveness. | |
Explanation | Use of the information, documents and data from the ECHA website is subject to the terms and conditions of this Legal Notice, and subject to other binding limitations provided for under applicable law, the information, documents and data made available on the ECHA website may be reproduced, distributed and/or used, totally or in part, for non-commercial purposes provided that ECHA is acknowledged as the source: "Source: European Chemicals Agency, http://echa.europa.eu/". Such acknowledgement must be included in each copy of the material. ECHA permits and encourages organisations and individuals to create links to the ECHA website under the following cumulative conditions: Links can only be made to webpages that provide a link to the Legal Notice page. | |
Retrosynthesis Analysis
AI-Powered Synthesis Planning: Our tool employs the Template_relevance Pistachio, Template_relevance Bkms_metabolic, Template_relevance Pistachio_ringbreaker, Template_relevance Reaxys, Template_relevance Reaxys_biocatalysis model, leveraging a vast database of chemical reactions to predict feasible synthetic routes.
One-Step Synthesis Focus: Specifically designed for one-step synthesis, it provides concise and direct routes for your target compounds, streamlining the synthesis process.
Accurate Predictions: Utilizing the extensive PISTACHIO, BKMS_METABOLIC, PISTACHIO_RINGBREAKER, REAXYS, REAXYS_BIOCATALYSIS database, our tool offers high-accuracy predictions, reflecting the latest in chemical research and data.
Strategy Settings
Precursor scoring | Relevance Heuristic |
---|---|
Min. plausibility | 0.01 |
Model | Template_relevance |
Template Set | Pistachio/Bkms_metabolic/Pistachio_ringbreaker/Reaxys/Reaxys_biocatalysis |
Top-N result to add to graph | 6 |
Feasible Synthetic Routes
Q & A
Q1: What is the molecular formula and weight of dichloromethane-d2?
A1: The molecular formula of dichloromethane-d2 is CD2Cl2, and its molecular weight is 86.95 g/mol.
Q2: How does deuteration affect the spectroscopic properties of dichloromethane?
A2: Deuteration significantly alters the NMR spectrum of dichloromethane. In 1H NMR, the signal from the CH2 group in dichloromethane (CH2Cl2) is absent in dichloromethane-d2 due to the replacement of hydrogen atoms with deuterium. This eliminates interference from the solvent signal, allowing for clearer observation of the analyte signals. []
Q3: Can you provide an example of how the chemical shifts of residual protons in dichloromethane-d2 are affected by the presence of salts?
A3: Research has shown that adding tetra-n-butylammonium chloride (TBACl) to dichloromethane-d2 causes a downfield shift in the residual proton signal. This shift is linearly dependent on the concentration of TBACl. []
Q4: Why is dichloromethane-d2 widely used as a solvent in NMR spectroscopy?
A4: Dichloromethane-d2 is a preferred solvent for NMR spectroscopy due to its several advantageous properties:
- Deuteration: Its deuterium atoms (2H) are NMR inactive in the 1H NMR frequency range, minimizing interference with analyte signals. []
Q5: How does dichloromethane-d2 affect the rate of olefin metathesis reactions catalyzed by certain ruthenium complexes?
A5: Studies on ring-closing olefin metathesis reactions, using complexes like [(η6-p-cymene)(PCy3)RuCl(CCCPh2)]OTf, have shown that reaction rates are generally slower in dichloromethane-d2 compared to benzene-d6. This suggests that solvent polarity can influence the catalytic activity of these ruthenium complexes. [, ]
Q6: Can you give an example of how dichloromethane-d2 is used to study reaction kinetics?
A6: Dichloromethane-d2 was used as a solvent in a study investigating the kinetics of fast hydrogen bond formation between pyridine and hexafluoroisopropanol. Using Coherent anti-Stokes Raman scattering (CARS) microspectroscopy, researchers were able to determine lower limits for the formation and dissociation rate constants of the hydrogen-bonded complex. []
Q7: Are there specific examples of reactions where dichloromethane-d2 is used to investigate reaction mechanisms?
A7: Yes, one example is the study of sulfonation reactions. Research on the sulfonation of allylsilanes with sulfur trioxide in dichloromethane-d2 provided direct NMR evidence for the formation of β-sultones as initial intermediates. This helped to elucidate the reaction mechanism and understand the selectivity of the sulfonation process. []
Q8: Can dichloromethane-d2 participate in reactions as more than just a solvent?
A8: While primarily used as a solvent, dichloromethane-d2 can participate in reactions under specific conditions. For example, in the presence of dimethyldioxirane-d6, dichloromethane-d2 can act as a source of ligands in reactions involving iron(III) tetramesitylporphyrin. This highlights the need to consider potential side reactions involving the solvent. []
Q9: How is dichloromethane-d2 utilized in computational chemistry studies?
A9: Dichloromethane-d2 serves as a model solvent in molecular dynamics simulations and quantum chemical calculations. Its properties and interactions with solutes can be simulated to understand various chemical phenomena, such as:
- Molecular Dynamics: Simulating molecular motions and interactions within dichloromethane-d2 can provide insights into solvation dynamics, conformational changes, and reaction mechanisms. []
- Vibrational Energy Relaxation: Studying the energy transfer between vibrationally excited molecules and dichloromethane-d2 can help understand solvent effects on vibrational relaxation processes. []
- Hydrogen Bonding: Investigating the nature and strength of hydrogen bonding interactions between solutes and dichloromethane-d2 can provide insights into molecular recognition and self-assembly processes. [, ]
Q10: Are there any applications of dichloromethane-d2 in materials science?
A10: Dichloromethane-d2 is used in materials science research, particularly in polymer chemistry. For instance, it was utilized in the characterization of supramolecular H-shaped (ter)polymers formed via multiple hydrogen bonding between Hamilton wedge (HW) functionalized block copolymers and cyanuric acid (CA) functionalized polymers. []
Avertissement et informations sur les produits de recherche in vitro
Veuillez noter que tous les articles et informations sur les produits présentés sur BenchChem sont destinés uniquement à des fins informatives. Les produits disponibles à l'achat sur BenchChem sont spécifiquement conçus pour des études in vitro, qui sont réalisées en dehors des organismes vivants. Les études in vitro, dérivées du terme latin "in verre", impliquent des expériences réalisées dans des environnements de laboratoire contrôlés à l'aide de cellules ou de tissus. Il est important de noter que ces produits ne sont pas classés comme médicaments et n'ont pas reçu l'approbation de la FDA pour la prévention, le traitement ou la guérison de toute condition médicale, affection ou maladie. Nous devons souligner que toute forme d'introduction corporelle de ces produits chez les humains ou les animaux est strictement interdite par la loi. Il est essentiel de respecter ces directives pour assurer la conformité aux normes légales et éthiques en matière de recherche et d'expérimentation.