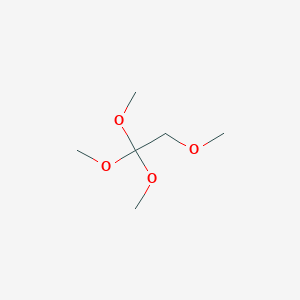
1,1,1,2-Tétraméthoxyéthane
- Cliquez sur DEMANDE RAPIDE pour recevoir un devis de notre équipe d'experts.
- Avec des produits de qualité à un prix COMPÉTITIF, vous pouvez vous concentrer davantage sur votre recherche.
Vue d'ensemble
Description
1,1,1,2-Tetramethoxyethane (TME) is an organic compound composed of four carbon atoms and eight hydrogen atoms connected to a single oxygen atom. It is a colorless, volatile liquid with a sweet odor. TME has a wide range of applications in organic chemistry, including as a solvent and a reagent for the synthesis of other organic compounds. It is also used in the production of pharmaceuticals, cosmetics, and other products. In the field of scientific research, TME has been used to study the mechanism of action of various drugs and to investigate the biochemical and physiological effects of certain compounds.
Applications De Recherche Scientifique
Applications de niche en nanotechnologie
Enfin, le composé trouve des applications de niche en nanotechnologie, en particulier dans la préparation de nanocomposites et de matériaux nanostructurés. Ses propriétés chimiques peuvent affecter l'auto-assemblage et l'alignement des nanoparticules.
Chacune de ces applications tire parti des propriétés chimiques uniques du 1,1,1,2-Tétraméthoxyéthane, démontrant sa polyvalence et son importance dans la recherche scientifique et les processus industriels. Les informations fournies ici sont basées sur les données disponibles jusqu'à ma dernière mise à jour en 2021 et des détails supplémentaires provenant de bases de données scientifiques . Pour les recherches et applications les plus récentes, consulter des bases de données spécialisées et des publications récentes fournirait les dernières informations.
Safety and Hazards
1,1,1,2-Tetramethoxyethane is classified as a potentially hazardous substance. It is recommended to avoid contact with skin and eyes, avoid formation of dust and aerosols, and use non-sparking tools . The safety information pictograms include GHS02 and GHS07, and the hazard statements include H226, H315, H319, and H335 .
Mécanisme D'action
, is also known as tetramethoxyethane . Here’s a breakdown of its key aspects:
Propriétés
IUPAC Name |
1,1,1,2-tetramethoxyethane |
Source
|
---|---|---|
Source | PubChem | |
URL | https://pubchem.ncbi.nlm.nih.gov | |
Description | Data deposited in or computed by PubChem | |
InChI |
InChI=1S/C6H14O4/c1-7-5-6(8-2,9-3)10-4/h5H2,1-4H3 |
Source
|
Source | PubChem | |
URL | https://pubchem.ncbi.nlm.nih.gov | |
Description | Data deposited in or computed by PubChem | |
InChI Key |
VPZFYLQMPOIPKH-UHFFFAOYSA-N |
Source
|
Source | PubChem | |
URL | https://pubchem.ncbi.nlm.nih.gov | |
Description | Data deposited in or computed by PubChem | |
Canonical SMILES |
COCC(OC)(OC)OC |
Source
|
Source | PubChem | |
URL | https://pubchem.ncbi.nlm.nih.gov | |
Description | Data deposited in or computed by PubChem | |
Molecular Formula |
C6H14O4 |
Source
|
Source | PubChem | |
URL | https://pubchem.ncbi.nlm.nih.gov | |
Description | Data deposited in or computed by PubChem | |
DSSTOX Substance ID |
DTXSID70187933 |
Source
|
Record name | 1,1,1,2-Tetramethoxyethane | |
Source | EPA DSSTox | |
URL | https://comptox.epa.gov/dashboard/DTXSID70187933 | |
Description | DSSTox provides a high quality public chemistry resource for supporting improved predictive toxicology. | |
Molecular Weight |
150.17 g/mol |
Source
|
Source | PubChem | |
URL | https://pubchem.ncbi.nlm.nih.gov | |
Description | Data deposited in or computed by PubChem | |
CAS RN |
34359-77-8 |
Source
|
Record name | 1,1,1,2-Tetramethoxyethane | |
Source | ChemIDplus | |
URL | https://pubchem.ncbi.nlm.nih.gov/substance/?source=chemidplus&sourceid=0034359778 | |
Description | ChemIDplus is a free, web search system that provides access to the structure and nomenclature authority files used for the identification of chemical substances cited in National Library of Medicine (NLM) databases, including the TOXNET system. | |
Record name | 1,1,1,2-Tetramethoxyethane | |
Source | EPA DSSTox | |
URL | https://comptox.epa.gov/dashboard/DTXSID70187933 | |
Description | DSSTox provides a high quality public chemistry resource for supporting improved predictive toxicology. | |
Record name | 1,1,1,2-tetramethoxyethane | |
Source | European Chemicals Agency (ECHA) | |
URL | https://echa.europa.eu/information-on-chemicals | |
Description | The European Chemicals Agency (ECHA) is an agency of the European Union which is the driving force among regulatory authorities in implementing the EU's groundbreaking chemicals legislation for the benefit of human health and the environment as well as for innovation and competitiveness. | |
Explanation | Use of the information, documents and data from the ECHA website is subject to the terms and conditions of this Legal Notice, and subject to other binding limitations provided for under applicable law, the information, documents and data made available on the ECHA website may be reproduced, distributed and/or used, totally or in part, for non-commercial purposes provided that ECHA is acknowledged as the source: "Source: European Chemicals Agency, http://echa.europa.eu/". Such acknowledgement must be included in each copy of the material. ECHA permits and encourages organisations and individuals to create links to the ECHA website under the following cumulative conditions: Links can only be made to webpages that provide a link to the Legal Notice page. | |
Avertissement et informations sur les produits de recherche in vitro
Veuillez noter que tous les articles et informations sur les produits présentés sur BenchChem sont destinés uniquement à des fins informatives. Les produits disponibles à l'achat sur BenchChem sont spécifiquement conçus pour des études in vitro, qui sont réalisées en dehors des organismes vivants. Les études in vitro, dérivées du terme latin "in verre", impliquent des expériences réalisées dans des environnements de laboratoire contrôlés à l'aide de cellules ou de tissus. Il est important de noter que ces produits ne sont pas classés comme médicaments et n'ont pas reçu l'approbation de la FDA pour la prévention, le traitement ou la guérison de toute condition médicale, affection ou maladie. Nous devons souligner que toute forme d'introduction corporelle de ces produits chez les humains ou les animaux est strictement interdite par la loi. Il est essentiel de respecter ces directives pour assurer la conformité aux normes légales et éthiques en matière de recherche et d'expérimentation.