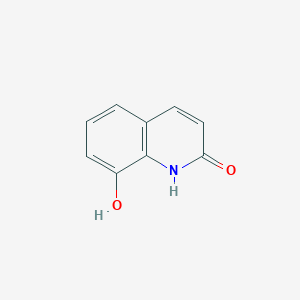
2,8-Quinolinediol
Vue d'ensemble
Description
2,8-Quinolinediol (C₉H₇NO₂; molecular weight: 161.16 g/mol; CAS: 15450-76-7) is a hydroxylated quinoline derivative characterized by two hydroxyl groups at positions 2 and 8 on the aromatic ring. It exists in tautomeric equilibrium with 8-hydroxyquinolin-2(1H)-one, a lactam form stabilized by intramolecular hydrogen bonding . Key properties include:
- Physical Properties: Melting point ~290°C, boiling point ~403.2°C, and solubility in DMSO but poor solubility in water or ethanol .
- Biosynthesis: Identified as a metabolite of 8-hydroxyquinoline-N-oxide in rabbits and as a product of quinoline degradation by Pseudomonas stutzeri .
- Applications: Used as a pharmaceutical intermediate for β₂-adrenergic receptor agonists (asthma therapeutics) and in toxicology studies to identify drug metabolites .
Its structural elucidation via HRMS and NMR confirms the planar aromatic system with hydroxyl groups influencing electron distribution and biological activity .
Méthodes De Préparation
Cyclocondensation of 2-Aminophenol with Acrolein
The most widely documented method for synthesizing 2,8-quinolinediol involves the cyclocondensation of 2-aminophenol with acrolein under acidic conditions . This reaction proceeds via a Friedländer-type mechanism, where the amine group of 2-aminophenol reacts with the α,β-unsaturated aldehyde (acrolein) to form the quinoline backbone.
Reaction Procedure
-
Acid Catalysis : A solution of 2-aminophenol (1 equivalent) in 6 N hydrochloric acid is heated under reflux.
-
Acrolein Addition : Acrolein (1.5 equivalents) is added dropwise to the reaction mixture, which is then stirred under reflux for 2 hours.
-
Neutralization : The mixture is cooled to room temperature, and the pH is adjusted to 7 using 6 N sodium hydroxide.
-
Extraction and Purification : The aqueous phase is extracted with ethyl acetate (3×), and the combined organic layers are dried over anhydrous MgSO₄. The crude product is purified via flash column chromatography (5–20% ethyl acetate in cyclohexane) .
Key Parameters
Parameter | Value/Description |
---|---|
Temperature | Reflux (~110°C for HCl solution) |
Reaction Time | 2 hours post-acrolein addition |
Solvent System | Ethyl acetate/cyclohexane |
Yield | ~34% (observed in analogous syntheses) |
This method’s moderate yield is attributed to competing side reactions, such as polymerization of acrolein or over-oxidation. Optimization studies suggest that controlled addition of acrolein and strict temperature monitoring improve selectivity .
Hydroxylation of 8-Hydroxyquinoline
An alternative route involves the direct hydroxylation of 8-hydroxyquinoline at the C2 position. While less commonly reported, this method leverages electrophilic aromatic substitution (EAS) mechanisms.
Mechanistic Insights
-
Electrophile Generation : Hydroxyl radicals (·OH) or metal-assisted oxidizing agents (e.g., H₂O₂/Fe²⁺) generate electrophilic species capable of attacking the electron-rich quinoline ring.
-
Regioselectivity : The C2 position is activated for substitution due to the directing effects of the existing C8 hydroxyl group and the nitrogen atom .
Limitations
-
Low Yield : Competing oxidation at other positions (e.g., C5 or C7) reduces regioselectivity.
-
Byproduct Formation : Over-oxidation may yield quinoline N-oxide derivatives, necessitating rigorous purification .
Technique | Findings |
---|---|
High-Resolution MS | m/z 161.16 [M+H]⁺ |
NMR Spectroscopy | δ 7.2–8.9 ppm (aromatic protons) |
UV-Vis Spectroscopy | λₘₐₓ = 335 nm (EtOH) |
Applications in Pharmaceutical Synthesis
While beyond direct preparation, this compound serves as a key intermediate for β₂-adrenergic receptor agonists like procaterol and indacaterol . Its synthetic utility underscores the importance of high-purity batches, achieved via:
Analyse Des Réactions Chimiques
Tautomerism and Structural Dynamics
2,8-Quinolinediol exists in equilibrium with its tautomer, 8-hydroxyquinolin-2(1H)-one, via keto-enol interconversion. This tautomerism is critical for its reactivity and interaction with biological targets .
Structural Features :
- Enol Form : Dominates in non-polar solvents, stabilized by intramolecular hydrogen bonding between the 2-OH and N atom.
- Keto Form : Prevalent in polar environments or under basic conditions, with a lactam structure.
Table 1 : Tautomeric equilibrium constants (K) in different solvents
Solvent | K (Enol:Keto) | Reference |
---|---|---|
Chloroform | 85:15 | |
Water (pH 7) | 40:60 |
Microbial Transformation
Pseudomonas stutzeri metabolizes quinoline via this compound as an intermediate. Key steps include:
- Hydroxylation : Quinoline → 2-hydroxyquinoline.
- Oxidative Ring Contraction : 2-hydroxyquinoline → this compound .
- Degradation : Further oxidation leads to aromatic ring cleavage, forming smaller metabolites (e.g., anthranilic acid) .
Table 2 : Metabolites in Pseudomonas stutzeri pathway
Substrate | Metabolite | Role in Pathway |
---|---|---|
Quinoline | 2-Hydroxyquinoline | Initial hydroxylation |
2-Hydroxyquinoline | This compound | Intermediate |
This compound | 8-Hydroxycoumarin | Ring contraction |
Sulfation and Conjugation
This compound undergoes sulfation at the 2-OH group in biological systems, forming This compound 2-sulfate (C₉H₅NO₆S). This reaction is catalyzed by sulfotransferases and enhances water solubility for excretion .
Reaction :
(PAPS = 3'-Phosphoadenosine-5'-phosphosulfate) .
Key Data :
Electrochemical Redox Activity
In supercapacitors, this compound-modified electrodes exhibit pseudocapacitance via reversible quinone-hydroquinone redox transitions .
Table 3 : Electrochemical performance in rGO-QD/MXene asymmetric supercapacitors
Parameter | Value | Reference |
---|---|---|
Specific Capacitance | 312 F/g at 1 A/g | |
Energy Density | 28.5 Wh/kg | |
Cycle Stability | 92% after 10k cycles |
Mechanism :
This Faradaic reaction occurs at the N-heteroatom and hydroxyl groups .
Aryl Hydrocarbon Receptor (AHR) Activation
This compound acts as a human-specific AHR agonist (EC₅₀ = 50 nM), binding to the receptor’s ligand-binding domain and inducing CYP1A1 transcription .
Key Findings :
- Species Specificity : 10-fold higher potency in human vs. mouse cells .
- Synergy with Cytokines : Co-treatment with IL-1β enhances IL6 expression 3-fold .
Binding Affinity :
Ligand | Kd (nM) | Cell Line |
---|---|---|
This compound | 12.4 | Human HepG2 |
TCDD (control) | 0.15 | Human HepG2 |
Oxidative Degradation
In advanced oxidation processes (AOPs), sulfate radicals (SO₄- ⁻) degrade this compound via:
- Hydroxyl Radical Attack : C-2 and C-8 hydroxylation.
- Ring Cleavage : Formation of oxalic acid and CO₂ .
Degradation Efficiency :
- Fe²⁺/ZVI/PS System : 92.9% removal in 15 min .
- Primary Byproducts : 8-Hydroxycoumarin, anthraquinone .
Metal Chelation
Though less studied than 8-hydroxyquinoline, this compound chelates transition metals (e.g., Fe³⁺, Cu²⁺) via its hydroxyl and N-heteroatom, forming stable complexes .
Proposed Structure :
Applications De Recherche Scientifique
Chemistry
2,8-Quinolinediol serves as a crucial building block for synthesizing various heterocyclic compounds. It is utilized as a standard in studies involving urinary metabolites and has been reported as an intermediate in the synthesis of other quinoline derivatives . Its ability to undergo oxidation and reduction reactions makes it valuable in organic synthesis.
Biology
In biological research, this compound is used to study metabolic pathways of quinolone derivatives in microorganisms such as Pseudomonas stutzeri. It has been identified as an Aryl Hydrocarbon Receptor (AHR) agonist, which plays a significant role in immune regulation and cellular metabolism. Studies have shown that it can induce the expression of cytochrome P450 enzymes (CYP1A1 and CYP1B1) in human cell lines at nanomolar concentrations .
Antimicrobial Properties
Research indicates that this compound exhibits antimicrobial properties, making it a candidate for further exploration in infection control .
Anti-inflammatory Effects
The compound may modulate inflammatory responses by enhancing IL-6 expression in gastrointestinal tissues when combined with cytokines .
Medicine
In medicinal chemistry, derivatives of quinoline compounds are important for synthesizing β2-adrenergic receptor agonists used in asthma treatment, such as procaterol and indacaterol . Ongoing research aims to elucidate the pharmacological potential of this compound and its derivatives.
Study on Microbiota-Derived Metabolites
A significant study highlighted the role of gut microbiota in producing 2,8-dihydroxyquinoline as an AHR ligand. In human cell lines, this metabolite exhibited full AHR agonist activity but showed reduced activity in mouse cells. The findings suggest that microbiota-derived metabolites like 2,8-DHQ contribute to AHR activation in the human gut .
Pharmacological Applications
Research has demonstrated that certain quinoline derivatives can inhibit Prion Protein formation in vitro and prolong incubation periods in experimental models of prion disease. While specific applications of this compound are still under investigation, its structural properties align with known pharmacologically active molecules .
Mécanisme D'action
The mechanism of action of 2,8-quinolinediol involves its interaction with various molecular targets and pathways. In biological systems, it can act as an inhibitor of certain enzymes or as a ligand for specific receptors. For instance, its derivatives are known to interact with β2-adrenergic receptors, leading to bronchodilation in asthma treatment . The exact pathways and molecular targets can vary depending on the specific application and derivative used.
Comparaison Avec Des Composés Similaires
Comparative Analysis with Structural Analogs
Positional Isomers: 2,4-Quinolinediol and 2,6-Quinolinediol
- 2,4-Quinolinediol: Found in Codonopsis tubers, it is associated with enhanced biosynthetic activity in plant tissues but lacks reported pharmacological roles .
- 2,6-Quinolinediol: Overexpressed in maize-fed rats, it exhibits antibiotic properties and regulates gut microbial diversity .
Methylated Analog: 2,8-Dimethylquinoline
2,8-Dimethylquinoline lacks hydroxyl groups, reducing its polarity and interaction with biological targets like the aryl hydrocarbon receptor (AHR) .
Other Dihydroxyquinolines: Quinoline-4,8-diol
Property | This compound | Quinoline-4,8-diol |
---|---|---|
Hydroxyl Positions | 2,8 | 4,8 |
Bioactivity | AHR agonist; DOX toxicity biomarker | Not well-characterized |
Detection | Urine, cow milk | Limited reports |
Research Findings and Functional Divergence
- AHR Activation: this compound derived from gut microbiota activates the aryl hydrocarbon receptor, contributing to intestinal homeostasis and immune regulation . In contrast, 2,6-quinolinediol primarily targets microbial communities without AHR involvement .
- Toxicology: this compound glucuronide (QGAC) is a biomarker for doxorubicin-induced toxicity, linked to disrupted tryptophan metabolism . No analogous role is reported for 2,4- or 2,6-isomers.
- Synthetic Utility: this compound serves as a precursor for β₂-adrenergic agonists, while its methylated analog (2,8-dimethylquinoline) is used in non-pharmaceutical applications .
Data Tables
Table 1: Physical and Chemical Properties
Activité Biologique
2,8-Quinolinediol, also known as 8-hydroxycarbostyril or 2,8-dihydroxyquinoline, is a compound belonging to the quinoline class, which has garnered attention due to its diverse biological activities. This article explores its biochemical properties, mechanisms of action, and potential applications in various fields.
Chemical Structure and Properties
The chemical formula of this compound is , and it features a bicyclic structure with hydroxyl groups at the 2 and 8 positions. This configuration allows for tautomerism between its keto and enol forms, influencing its reactivity and biological interactions.
Property | Value |
---|---|
Molecular Weight | 161.16 g/mol |
IUPAC Name | 8-hydroxy-1,2-dihydroquinolin-2-one |
CAS Number | 15450-76-7 |
Solubility | 5.14 g/L |
This compound exhibits its biological effects primarily through interactions with various biomolecules, including enzymes and receptors. Notably, it acts as an agonist of the Aryl Hydrocarbon Receptor (AHR), which plays a crucial role in immune regulation and cellular metabolism. Research has shown that it can significantly induce the expression of cytochrome P450 enzymes (CYP1A1 and CYP1B1) in human cell lines at nanomolar concentrations .
Key Mechanisms:
- AHR Activation : It binds to AHR, leading to the transcriptional activation of target genes involved in xenobiotic metabolism and immune responses .
- Enzyme Interaction : The compound influences various metabolic pathways by interacting with specific enzymes, thereby affecting cellular functions such as signal transduction and gene expression .
Biological Activities
The biological activities of this compound extend across several domains:
1. Antimicrobial Properties
2. Anti-inflammatory Effects
- By activating AHR, this compound may modulate inflammatory responses in gastrointestinal tissues. Studies indicate that it can enhance IL-6 expression when combined with cytokines, suggesting a potential role in inflammatory modulation .
3. Cancer Research
- The compound's ability to influence metabolic pathways related to AHR activation positions it as a candidate for further research in cancer biology. Its effects on CYP enzymes may be relevant for understanding drug metabolism and resistance mechanisms in cancer therapy.
Study on Microbiota-Derived Metabolites
A significant study highlighted the role of microbiota in producing 2,8-dihydroxyquinoline as an AHR ligand. In human cell lines, this metabolite exhibited full AHR agonist activity but showed reduced activity in mouse cells. The findings suggest that gut microbiota may influence host immune responses through metabolites like 2,8-DHQ .
Pharmacological Applications
Research indicates that derivatives of quinoline compounds are utilized in synthesizing β2-adrenergic receptor agonists for asthma treatment. While specific applications of this compound are still under investigation, its structural properties align with those of known pharmacologically active molecules.
Q & A
Basic Research Questions
Q. How is 2,8-Quinolinediol structurally characterized in metabolic studies?
- Methodological Answer : Structural elucidation employs high-resolution mass spectrometry (HRMS) for molecular weight confirmation (161.16 g/mol) and nuclear magnetic resonance (NMR) spectroscopy (1H, 13C, and 1H×13C) to identify hydroxyl groups at C2 and C8. The keto-enol tautomerism (e.g., 8-hydroxyquinolin-2(1H)-one as a cis-isomer) is resolved via NMR chemical shifts .
Q. What experimental protocols are recommended for handling this compound in laboratory settings?
- Methodological Answer : Due to its irritant properties (eye/skin irritation Category 2), use N95 masks, gloves, and goggles. Store at -20°C for stability, and dissolve in DMSO (>38.8 mg/mL) for in vitro studies. Avoid aqueous or ethanol solutions due to insolubility .
Q. How is this compound used as a reference standard in LC-MS metabolomics?
- Methodological Answer : As a urinary metabolite marker, it is spiked into samples at known concentrations (e.g., 1–50 µM) for calibration. Ultra-performance LC-MS (UPLC-MS) with C18 columns and negative ionization mode optimizes detection .
Q. What are the key physicochemical properties influencing its reactivity?
- Methodological Answer : The phenolic hydroxyl groups enable redox reactions, chelation, and participation in hydrogen bonding. Its melting point (290°C) and stability under inert conditions make it suitable for high-temperature syntheses .
Advanced Research Questions
Q. How does this compound contribute to doxorubicin (DOX) toxicity studies?
- Methodological Answer : In rat models, urinary this compound glucuronide (QGAC) decreases post-DOX administration, reflecting disrupted gut microbiota metabolism. Systems toxicology approaches integrate metabolomics (UPLC-MS) and pathway analysis (MetaCore) to link QGAC to oxidative stress and renal dysfunction .
Q. What contradictory evidence exists regarding its metabolic origin in mammalian systems?
- Methodological Answer : While detected in rat urine, this compound is absent in corn diets, suggesting gut microbiota-mediated transformation of precursors like quinoline. Contrasting studies attribute its presence to Pseudomonas stutzeri degradation pathways .
Q. How can DFT calculations and X-ray crystallography resolve tautomeric ambiguities?
- Methodological Answer : Density functional theory (DFT) optimizes molecular geometries to predict tautomer stability (keto vs. enol forms). X-ray diffraction confirms solid-state structures, revealing intermolecular hydrogen bonding patterns .
Q. What role does this compound play in β2-adrenergic receptor agonist synthesis?
- Methodological Answer : As a starting reagent, it undergoes regioselective alkylation or acylation at C2/C8 hydroxyls. Reaction conditions (e.g., anhydrous DMF, 60°C) and protecting groups (e.g., tert-butyldimethylsilyl) improve yield .
Q. How is this compound implicated in blood dialysis-associated cognitive decline?
- Methodological Answer : Cohort studies correlate elevated serum levels with mild cognitive impairment in dialysis patients. Targeted LC-MS quantifies its accumulation, while in vitro blood-brain barrier models assess neurotoxicity via ROS generation assays .
Q. Data Contradictions and Analytical Challenges
Q. Why do metabolite levels of this compound vary across species and dietary conditions?
- Methodological Answer : In weaned piglets, its fecal concentration increases 15.3-fold (FC=15.31) with specific feed additives, suggesting diet-gut microbiome interactions. However, interspecies differences in cytochrome P450 activity alter metabolic rates .
Q. How to address discrepancies in its reported solubility and stability?
- Methodological Answer : While insoluble in H2O/EtOH, DMSO enhances solubility for cell-based assays. Conflicting stability data (e.g., RT vs. -20°C storage) necessitate empirical validation via accelerated degradation studies (40°C/75% RH for 4 weeks) .
Q. Methodological Optimization
Q. What strategies improve synthetic yields of this compound?
- Methodological Answer : Optimize the acetylation of quinoline-8-ol-1-oxide in acetic anhydride (90°C, 4 hr), followed by neutralization with NH4OH. Recrystallization in propylene glycol/petroleum ether achieves >98% purity .
Q. How to mitigate interference from isomers in analytical workflows?
- Methodological Answer : Chromatographic separation (e.g., HILIC columns) distinguishes this compound from 2,4- and 4,8-isomers. MS/MS fragmentation patterns (e.g., m/z 161→143 for hydroxyl loss) confirm identity .
Q. Emerging Applications
Q. Can this compound serve as a fluorescent probe for metal ion detection?
- Methodological Answer : Functionalization at C2/C8 with amide groups (e.g., 8-amidoquinoline derivatives) enhances metal chelation. Fluorescence quenching assays (λex=335 nm) quantify Fe³⁺/Cu²⁺ binding in environmental samples .
Q. What advanced models explore its ecotoxicological impact?
Propriétés
IUPAC Name |
8-hydroxy-1H-quinolin-2-one | |
---|---|---|
Source | PubChem | |
URL | https://pubchem.ncbi.nlm.nih.gov | |
Description | Data deposited in or computed by PubChem | |
InChI |
InChI=1S/C9H7NO2/c11-7-3-1-2-6-4-5-8(12)10-9(6)7/h1-5,11H,(H,10,12) | |
Source | PubChem | |
URL | https://pubchem.ncbi.nlm.nih.gov | |
Description | Data deposited in or computed by PubChem | |
InChI Key |
ZXZKYYHTWHJHFT-UHFFFAOYSA-N | |
Source | PubChem | |
URL | https://pubchem.ncbi.nlm.nih.gov | |
Description | Data deposited in or computed by PubChem | |
Canonical SMILES |
C1=CC2=C(C(=C1)O)NC(=O)C=C2 | |
Source | PubChem | |
URL | https://pubchem.ncbi.nlm.nih.gov | |
Description | Data deposited in or computed by PubChem | |
Molecular Formula |
C9H7NO2 | |
Source | PubChem | |
URL | https://pubchem.ncbi.nlm.nih.gov | |
Description | Data deposited in or computed by PubChem | |
DSSTOX Substance ID |
DTXSID70165650 | |
Record name | 2,8-Dihydroxyquinoline | |
Source | EPA DSSTox | |
URL | https://comptox.epa.gov/dashboard/DTXSID70165650 | |
Description | DSSTox provides a high quality public chemistry resource for supporting improved predictive toxicology. | |
Molecular Weight |
161.16 g/mol | |
Source | PubChem | |
URL | https://pubchem.ncbi.nlm.nih.gov | |
Description | Data deposited in or computed by PubChem | |
CAS No. |
15450-76-7 | |
Record name | 8-Hydroxy-1H-quinolin-2-one | |
Source | CAS Common Chemistry | |
URL | https://commonchemistry.cas.org/detail?cas_rn=15450-76-7 | |
Description | CAS Common Chemistry is an open community resource for accessing chemical information. Nearly 500,000 chemical substances from CAS REGISTRY cover areas of community interest, including common and frequently regulated chemicals, and those relevant to high school and undergraduate chemistry classes. This chemical information, curated by our expert scientists, is provided in alignment with our mission as a division of the American Chemical Society. | |
Explanation | The data from CAS Common Chemistry is provided under a CC-BY-NC 4.0 license, unless otherwise stated. | |
Record name | 2,8-Dihydroxyquinoline | |
Source | ChemIDplus | |
URL | https://pubchem.ncbi.nlm.nih.gov/substance/?source=chemidplus&sourceid=0015450767 | |
Description | ChemIDplus is a free, web search system that provides access to the structure and nomenclature authority files used for the identification of chemical substances cited in National Library of Medicine (NLM) databases, including the TOXNET system. | |
Record name | 2,8-Quinolinediol | |
Source | DTP/NCI | |
URL | https://dtp.cancer.gov/dtpstandard/servlet/dwindex?searchtype=NSC&outputformat=html&searchlist=108383 | |
Description | The NCI Development Therapeutics Program (DTP) provides services and resources to the academic and private-sector research communities worldwide to facilitate the discovery and development of new cancer therapeutic agents. | |
Explanation | Unless otherwise indicated, all text within NCI products is free of copyright and may be reused without our permission. Credit the National Cancer Institute as the source. | |
Record name | 2,8-Dihydroxyquinoline | |
Source | EPA DSSTox | |
URL | https://comptox.epa.gov/dashboard/DTXSID70165650 | |
Description | DSSTox provides a high quality public chemistry resource for supporting improved predictive toxicology. | |
Record name | 8-HYDROXYCARBOSTYRIL | |
Source | FDA Global Substance Registration System (GSRS) | |
URL | https://gsrs.ncats.nih.gov/ginas/app/beta/substances/466PKO4UBM | |
Description | The FDA Global Substance Registration System (GSRS) enables the efficient and accurate exchange of information on what substances are in regulated products. Instead of relying on names, which vary across regulatory domains, countries, and regions, the GSRS knowledge base makes it possible for substances to be defined by standardized, scientific descriptions. | |
Explanation | Unless otherwise noted, the contents of the FDA website (www.fda.gov), both text and graphics, are not copyrighted. They are in the public domain and may be republished, reprinted and otherwise used freely by anyone without the need to obtain permission from FDA. Credit to the U.S. Food and Drug Administration as the source is appreciated but not required. | |
Record name | 2,8-Quinolinediol | |
Source | Human Metabolome Database (HMDB) | |
URL | http://www.hmdb.ca/metabolites/HMDB0240311 | |
Description | The Human Metabolome Database (HMDB) is a freely available electronic database containing detailed information about small molecule metabolites found in the human body. | |
Explanation | HMDB is offered to the public as a freely available resource. Use and re-distribution of the data, in whole or in part, for commercial purposes requires explicit permission of the authors and explicit acknowledgment of the source material (HMDB) and the original publication (see the HMDB citing page). We ask that users who download significant portions of the database cite the HMDB paper in any resulting publications. | |
Synthesis routes and methods
Procedure details
Retrosynthesis Analysis
AI-Powered Synthesis Planning: Our tool employs the Template_relevance Pistachio, Template_relevance Bkms_metabolic, Template_relevance Pistachio_ringbreaker, Template_relevance Reaxys, Template_relevance Reaxys_biocatalysis model, leveraging a vast database of chemical reactions to predict feasible synthetic routes.
One-Step Synthesis Focus: Specifically designed for one-step synthesis, it provides concise and direct routes for your target compounds, streamlining the synthesis process.
Accurate Predictions: Utilizing the extensive PISTACHIO, BKMS_METABOLIC, PISTACHIO_RINGBREAKER, REAXYS, REAXYS_BIOCATALYSIS database, our tool offers high-accuracy predictions, reflecting the latest in chemical research and data.
Strategy Settings
Precursor scoring | Relevance Heuristic |
---|---|
Min. plausibility | 0.01 |
Model | Template_relevance |
Template Set | Pistachio/Bkms_metabolic/Pistachio_ringbreaker/Reaxys/Reaxys_biocatalysis |
Top-N result to add to graph | 6 |
Feasible Synthetic Routes
Avertissement et informations sur les produits de recherche in vitro
Veuillez noter que tous les articles et informations sur les produits présentés sur BenchChem sont destinés uniquement à des fins informatives. Les produits disponibles à l'achat sur BenchChem sont spécifiquement conçus pour des études in vitro, qui sont réalisées en dehors des organismes vivants. Les études in vitro, dérivées du terme latin "in verre", impliquent des expériences réalisées dans des environnements de laboratoire contrôlés à l'aide de cellules ou de tissus. Il est important de noter que ces produits ne sont pas classés comme médicaments et n'ont pas reçu l'approbation de la FDA pour la prévention, le traitement ou la guérison de toute condition médicale, affection ou maladie. Nous devons souligner que toute forme d'introduction corporelle de ces produits chez les humains ou les animaux est strictement interdite par la loi. Il est essentiel de respecter ces directives pour assurer la conformité aux normes légales et éthiques en matière de recherche et d'expérimentation.