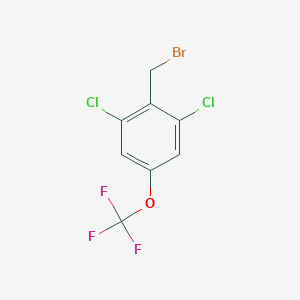
2,6-Dichloro-4-(trifluoromethoxy)benzyl bromide
- Cliquez sur DEMANDE RAPIDE pour recevoir un devis de notre équipe d'experts.
- Avec des produits de qualité à un prix COMPÉTITIF, vous pouvez vous concentrer davantage sur votre recherche.
Vue d'ensemble
Description
“2,6-Dichloro-4-(trifluoromethoxy)benzyl bromide” is a synthetic intermediate . It has a molecular formula of C8H4BrCl2F3O and a molecular weight of 323.92 .
Synthesis Analysis
This compound can be synthesized via Friedel-Crafts polymerization using aluminum chloride as a catalyst . It is used to prepare (nitro)[(trifluoromethoxy)benzyloxy]dihydroimidazo[2,1-b][1,3]oxazines with antitubercular activities .Molecular Structure Analysis
The molecular structure of this compound includes a benzyl group attached to a trifluoromethoxy group and two chlorine atoms . The exact structure can be found in various chemical databases .Chemical Reactions Analysis
As a synthetic intermediate, this compound is involved in various chemical reactions. For instance, it is used in the synthesis of bioreductive drugs . More specific reactions would depend on the context of the synthesis.Physical And Chemical Properties Analysis
This compound is a liquid at room temperature with a density of 1.594 g/mL at 25 °C . It has a boiling point of 82-84 °C/10 mmHg . The compound’s refractive index is 1.48 .Applications De Recherche Scientifique
Synthesis of Bioreductive Drugs
2,6-Dichloro-4-(trifluoromethoxy)benzyl bromide: is utilized in the synthesis of bioreductive drugs such as PA-824 . PA-824 is a nitroimidazo-oxazine derivative that exhibits promising activity against Mycobacterium tuberculosis . The compound acts as a prodrug that is reductively activated in anaerobic conditions, which are typical of tuberculosis lesions.
Safety and Hazards
Mécanisme D'action
Target of Action
The primary target of 2,6-Dichloro-4-(trifluoromethoxy)benzyl bromide is involved in the Suzuki–Miyaura (SM) cross-coupling reaction . This reaction is a widely-applied transition metal catalyzed carbon–carbon bond forming reaction .
Mode of Action
The compound interacts with its targets through a process known as transmetalation . In the Suzuki–Miyaura coupling reaction, oxidative addition occurs with formally electrophilic organic groups, whereby palladium becomes oxidized through its donation of electrons to form the new Pd–C bond . Transmetalation occurs with formally nucleophilic organic groups, which are transferred from boron to palladium .
Biochemical Pathways
The compound is involved in the Suzuki–Miyaura coupling reaction, which is a key biochemical pathway in organic synthesis . The downstream effects of this pathway include the formation of carbon–carbon bonds, which are fundamental in the construction of complex organic molecules .
Result of Action
The compound’s action results in the formation of carbon–carbon bonds via the Suzuki–Miyaura coupling reaction . This can lead to the synthesis of complex organic molecules, which can have various applications in fields such as pharmaceuticals .
Action Environment
The efficacy and stability of 2,6-Dichloro-4-(trifluoromethoxy)benzyl bromide can be influenced by various environmental factors. For instance, the Suzuki–Miyaura coupling reaction is known to be exceptionally mild and functional group tolerant . Therefore, the reaction conditions can significantly impact the compound’s action. Additionally, the compound’s stability may be affected by factors such as temperature and pH .
Propriétés
IUPAC Name |
2-(bromomethyl)-1,3-dichloro-5-(trifluoromethoxy)benzene |
Source
|
---|---|---|
Source | PubChem | |
URL | https://pubchem.ncbi.nlm.nih.gov | |
Description | Data deposited in or computed by PubChem | |
InChI |
InChI=1S/C8H4BrCl2F3O/c9-3-5-6(10)1-4(2-7(5)11)15-8(12,13)14/h1-2H,3H2 |
Source
|
Source | PubChem | |
URL | https://pubchem.ncbi.nlm.nih.gov | |
Description | Data deposited in or computed by PubChem | |
InChI Key |
ZHLKYFQGUSHCIH-UHFFFAOYSA-N |
Source
|
Source | PubChem | |
URL | https://pubchem.ncbi.nlm.nih.gov | |
Description | Data deposited in or computed by PubChem | |
Canonical SMILES |
C1=C(C=C(C(=C1Cl)CBr)Cl)OC(F)(F)F |
Source
|
Source | PubChem | |
URL | https://pubchem.ncbi.nlm.nih.gov | |
Description | Data deposited in or computed by PubChem | |
Molecular Formula |
C8H4BrCl2F3O |
Source
|
Source | PubChem | |
URL | https://pubchem.ncbi.nlm.nih.gov | |
Description | Data deposited in or computed by PubChem | |
Molecular Weight |
323.92 g/mol |
Source
|
Source | PubChem | |
URL | https://pubchem.ncbi.nlm.nih.gov | |
Description | Data deposited in or computed by PubChem | |
Product Name |
2,6-Dichloro-4-(trifluoromethoxy)benzyl bromide |
Avertissement et informations sur les produits de recherche in vitro
Veuillez noter que tous les articles et informations sur les produits présentés sur BenchChem sont destinés uniquement à des fins informatives. Les produits disponibles à l'achat sur BenchChem sont spécifiquement conçus pour des études in vitro, qui sont réalisées en dehors des organismes vivants. Les études in vitro, dérivées du terme latin "in verre", impliquent des expériences réalisées dans des environnements de laboratoire contrôlés à l'aide de cellules ou de tissus. Il est important de noter que ces produits ne sont pas classés comme médicaments et n'ont pas reçu l'approbation de la FDA pour la prévention, le traitement ou la guérison de toute condition médicale, affection ou maladie. Nous devons souligner que toute forme d'introduction corporelle de ces produits chez les humains ou les animaux est strictement interdite par la loi. Il est essentiel de respecter ces directives pour assurer la conformité aux normes légales et éthiques en matière de recherche et d'expérimentation.