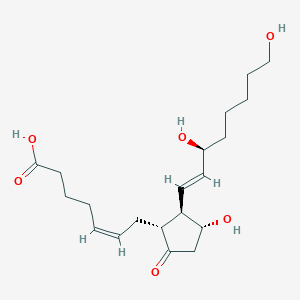
20-Hydroxy-PGE2
Vue d'ensemble
Description
20-Hydroxy-prostaglandin E2 (20-Hydroxy-PGE2) is a cytochrome P-450-derived metabolite of prostaglandin E2 (PGE2). It plays significant roles in lipid metabolism, adipogenesis, and disease pathophysiology. Studies demonstrate its involvement in adipocyte hypertrophy, where 100–1,000 nM concentrations increase lipid droplet size by 2- to 3-fold . Additionally, this compound serves as a biomarker for diabetic nephropathy (DN), with elevated levels observed in urine samples from patients with pyuria or hematuria . Its stability in clinical samples is temperature-dependent, requiring prompt freezing for accurate quantification .
Méthodes De Préparation
Chemical Synthesis Strategies
Oxidation of PGF2α Derivatives
A patented green synthesis method for PGE2 involves oxidizing 11,15-di-protected PGF2α using pyridine sulfur trioxide (Py·SO3) in dimethyl sulfoxide (DMSO) . This reaction converts secondary alcohols to ketones, yielding protected PGE2, which is subsequently deprotected using citric acid (Table 1). Adapting this method to introduce a 20-hydroxy group would require starting with a PGF2α analog pre-functionalized at position 20. For example, introducing a tert-butyldimethylsilyl (TBS)-protected hydroxyl group at C20 prior to oxidation could enable post-synthetic deprotection to yield 20-hydroxy-PGE2 .
Table 1: Key Reaction Conditions for PGF2α to PGE2 Conversion
Step | Reagents/Conditions | Yield (%) | Purity (%) |
---|---|---|---|
Oxidation | Py·SO3, DMSO, triethylamine | 73–78 | >90 |
Deprotection | 1% HCl in MeOH | 80–83 | 95 |
Crystallization | Ethyl acetate/n-hexane | 65–70 | 99 |
Tail-Modification of PGE2 Analogs
The synthesis of PGE2 ethanolamide (PGE2-EA) and glycerol ester analogs demonstrates the feasibility of modifying the omega-chain (positions 17–20) of PGE2 . Using Horner–Wadsworth–Emmons reactions, researchers installed azido and isothiocyanato functionalities at the terminal carbon, achieving yields of 73–78% for critical coupling steps . Applying similar strategies, a hydroxyl group could be introduced at C20 via Mitsunobu azidation or peptide coupling, followed by deprotection (Figure 1).
Semi-Synthetic Approaches
Enzymatic Hydroxylation Followed by Chemical Refinement
Combining enzymatic and chemical methods offers a promising route. For instance, incubating 20-hydroxy-arachidonic acid with ram vesicular gland microsomes and glutathione could yield 20-hydroxy-PGH2, which prostaglandin E synthases might then isomerize to this compound . Subsequent chemical protection (e.g., TIPS or TBS groups) and purification steps would enhance stability and yield.
Lipase-Mediated Deprotection
In the synthesis of 2-PGE2-G, Candida rugosa lipase selectively hydrolyzes triglyceride intermediates to yield glycerol esters . This method could be adapted to deprotect 20-O-acetyl-PGE2, leveraging lipase’s regioselectivity to retain the 20-hydroxy group.
Challenges and Optimization
Stereochemical Control
Introducing a hydroxyl group at C20 without epimerizing adjacent chiral centers (e.g., C15) remains a key challenge. Corey’s lactone-based synthesis, which uses TIPS and TBS protecting groups, provides a model for maintaining stereochemical integrity during oxidation .
Yield Limitations
Current methods for related prostaglandins report yields of 65–70% after crystallization . Scaling this compound synthesis will require optimizing solvent systems (e.g., ethyl acetate/n-hexane) and minimizing side reactions during deprotection.
Analyse Des Réactions Chimiques
ω-Oxidation at C-20 via Cytochrome P450 Enzymes
20-Hydroxy-PGE2 is formed through CYP450-mediated ω-oxidation of PGE2. This reaction introduces a hydroxyl group at the terminal carbon (C-20) of the fatty acid side chain.
- Enzymes : CYP4F and CYP4A subfamilies are implicated in this reaction .
- Substrates : PGE2 is the primary substrate, with higher efficiency compared to other prostaglandins (e.g., PGF2α) .
- Cofactors : NADPH is required for CYP450 activity .
- Reaction :
Key Data :
- Ram seminal vesicle microsomes convert PGE2 to this compound at 5–10% efficiency compared to PGE2’s conversion to other metabolites .
- Human seminal vesicles produce 19-Hydroxy-PGE2 as a positional isomer, highlighting species-specific CYP450 activity .
β-Oxidation of the ω-Side Chain
Following ω-oxidation, this compound undergoes β-oxidation , shortening the carbon chain by up to four carbons .
- Enzymes : Acyl-CoA dehydrogenases and thiolases.
- Intermediates : Successive removal of two-carbon units yields metabolites like 16-Hydroxy-PGE2 and 14-Hydroxy-PGE2 .
- Reaction Pathway :
Research Findings :
- β-oxidation is a dominant metabolic pathway in hepatic and renal tissues, facilitating rapid clearance of this compound .
Enzymatic Reduction and Isomerization
This compound can undergo reduction or epimerization under specific conditions:
- Reduction :
- Isomerization :
Conjugation Reactions
This compound undergoes glucuronidation and sulfation to enhance water solubility for excretion:
- Enzymes : UDP-glucuronosyltransferases (UGTs) and sulfotransferases (SULTs) .
- Products : Glucuronide and sulfate conjugates detected in urine and plasma .
Research Highlights
- Substrate Specificity :
- Pharmacological Implications :
- Species Variability :
Applications De Recherche Scientifique
Role in Tumorigenesis
20-Hydroxy-PGE2 has been implicated in cancer biology, particularly in how it modulates tumor growth and metastasis. Studies indicate that it interacts with specific receptors (EP receptors) that are often overexpressed in various cancers, including prostate, breast, and colorectal cancers. The activation of these receptors can lead to enhanced cell proliferation and invasion capabilities .
- Mechanisms of Action :
- EP Receptor Activation : this compound binds to EP receptors, triggering multiple signaling pathways associated with cancer progression such as the PI3K/AKT and β-catenin pathways. These pathways are crucial for regulating genes involved in cell cycle progression and survival .
- Inhibition of Apoptosis : The compound may also play a role in inhibiting apoptosis in tumor cells, contributing to tumor survival and resistance to therapy.
Clinical Implications
Targeting the pathways influenced by this compound could provide new therapeutic strategies for cancer treatment. For instance, antagonists of EP receptors are being investigated for their potential to inhibit tumor growth and metastasis .
Inflammation and Immune Response
This compound exhibits anti-inflammatory properties, influencing immune responses in various contexts. It has been shown to modulate the activity of immune cells such as macrophages and T cells.
- Mechanisms :
- Regulation of Cytokine Production : It can influence the production of pro-inflammatory cytokines, thereby playing a role in inflammatory diseases .
- Effects on Macrophage Function : Research indicates that it can alter macrophage polarization, potentially shifting them from a pro-inflammatory M1 phenotype to an anti-inflammatory M2 phenotype .
Potential Therapeutic Uses
Given its dual role in inflammation—both promoting and inhibiting—it may serve as a target for developing drugs aimed at treating inflammatory diseases or conditions characterized by dysregulated immune responses.
Metabolic Regulation
Recent studies have highlighted the role of this compound in metabolic processes:
- Lipid Metabolism : It has been observed that this compound induces lipid accumulation and promotes adipocyte differentiation in human mesenchymal stem cells. This suggests its involvement in metabolic disorders such as obesity and diabetes .
- Impact on Insulin Sensitivity : There is emerging evidence that it may influence insulin signaling pathways, further linking it to metabolic health .
Neurobiology
The compound also shows promise in neurobiological applications:
- Neuroprotective Effects : Some studies suggest that this compound may have neuroprotective effects against neurodegenerative diseases by modulating neuroinflammatory processes .
- Behavioral Implications : Research into its effects on behavior indicates potential roles in mood regulation and cognitive functions, although more studies are needed to elucidate these connections fully.
Data Summary Table
Application Area | Mechanism of Action | Potential Therapeutic Use |
---|---|---|
Cancer | EP receptor activation; inhibition of apoptosis | Cancer treatment via EP antagonists |
Inflammation | Modulation of cytokine production; macrophage function | Anti-inflammatory drugs |
Metabolic Regulation | Induction of lipid accumulation; influence on insulin | Treatments for obesity and diabetes |
Neurobiology | Neuroprotective effects; modulation of neuroinflammation | Potential treatments for neurodegenerative diseases |
Mécanisme D'action
20-hydroxy Prostaglandin E2 exerts its effects by binding to specific prostaglandin receptors, primarily the EP2 receptor. This binding activates intracellular signaling pathways, including the cyclic AMP pathway, leading to various physiological responses such as smooth muscle relaxation and modulation of inflammatory processes . The cytochrome P450 enzymes play a crucial role in its metabolism, converting Prostaglandin E2 to 20-hydroxy Prostaglandin E2 .
Comparaison Avec Des Composés Similaires
Comparison with Structurally Similar Compounds
19-Hydroxy-PGE2
- Structural Difference : Hydroxylation occurs at carbon 19 (C19) instead of C20.
- Biological Role: Found in mammalian seminal fluid, its levels vary due to cytochrome P-450 polymorphisms in rams.
- Analytical Challenge : Co-elutes with 20-Hydroxy-PGE2 in LC-MS/MS, necessitating advanced spectral separation techniques .
13,14-Dihydro-15-Keto-PGE2
- Structural Difference : Features saturation of the C13–C14 double bond and a keto group at C13.
- Biological Role : Elevated in advanced stages of DN, contrasting with this compound, which rises earlier in pyuria .
- Analytical Advantage : Distinct retention times in LC-MS allow easier quantification compared to this compound .
16,16-Dimethyl-PGE2
- Structural Difference : Synthetic analog with methyl groups at C14.
- Functional Role : Designed to resist enzymatic degradation by 15-hydroxyprostaglandin dehydrogenase (15-PGDH), enhancing metabolic stability. Unlike this compound, it is used experimentally to prolong PGE2 signaling .
Functional Comparison with Related Prostaglandins
PGE2 (Parent Compound)
- Adipogenesis : PGE2 promotes cancer stem cell (CSC) expansion in colorectal cancer (CRC), while this compound levels are paradoxically lower in CRC patients .
- Analytical Differentiation : PGE2 lacks hydroxylation at C20, enabling straightforward separation via LC-MS .
5-Hydroxy-PGE2
- Biosynthetic Pathway : Generated via crossover between 5-lipoxygenase and cyclooxygenase-2 (COX-2), unlike this compound, which is COX-2-dependent .
- Functional Overlap : Both compounds regulate inflammatory pathways but differ in tissue-specific effects.
Research Findings and Clinical Implications
- Adipogenesis : this compound stimulates adipocyte hypertrophy via COX-2, an effect antagonized by PGE2 .
- Diabetic Nephropathy: Urinary this compound levels increase in pyuria and hematuria, making it a candidate biomarker for early DN diagnosis .
- Cancer : Lower serum levels in CRC patients suggest a divergent role from PGE2 in tumor progression .
Analytical Challenges and Methodological Considerations
- Co-Elution Issues: this compound and 19-Hydroxy-PGE2 cannot be separated using standard LC-MS/MS methods, requiring compound-specific fragment ions for quantification .
- Sample Stability : Urinary this compound levels increase at room temperature, necessitating immediate freezing to prevent artifactually elevated readings .
Activité Biologique
20-Hydroxy-PGE2 (20-hydroxylated prostaglandin E2) is a metabolite of prostaglandin E2 (PGE2) known for its diverse biological activities. This article reviews the synthesis, biological functions, and implications of this compound in various physiological and pathological contexts, supported by case studies and research findings.
Synthesis of this compound
This compound is formed through the enzymatic action of specific hydroxylases that convert PGE2 into its 20-hydroxylated form. Research has shown that microsomes derived from the ampullary mucosa of ram ductus deferens can metabolize PGE2 to this compound at a rate of approximately 7.1 pmol min mg of protein . This conversion is facilitated by the presence of NADPH and can be analyzed using techniques such as gas chromatography-mass spectrometry (GC-MS) and high-performance liquid chromatography (HPLC) for quantification .
Biological Functions
Pro-inflammatory and Anti-inflammatory Roles:
The biological activity of this compound is context-dependent, exhibiting both pro-inflammatory and anti-inflammatory properties. It plays a crucial role in modulating immune responses, particularly in conditions like type 1 diabetes mellitus (T1DM), where it influences T-cell polarization. High concentrations of PGE2 have been shown to suppress Th1 cells while promoting Th2 differentiation, indicating a potential therapeutic target for managing inflammation .
Influence on Cancer:
In cancer biology, this compound is implicated in tumor progression through its interaction with EP receptors (EP1-EP4). For instance, signaling through the EP2 receptor has been associated with increased expression of COX-2 in tumor tissues, enhancing tumor growth and metastasis . Additionally, it has been observed that antagonists targeting EP receptors can mitigate chronic inflammation in tumor microenvironments, suggesting a dual role in cancer development and treatment .
Case Studies
Case Study 1: Type 1 Diabetes Mellitus
In a study focusing on the PTGS2/COX2-PGE2 signaling cascade in T1DM, it was found that PGE2 promotes an anti-inflammatory environment at high concentrations. This effect was mediated through its binding to EP receptors expressed on T-cells, highlighting the complexity of PGE2's role in autoimmune diseases .
Case Study 2: Cancer Progression
Research has demonstrated that elevated levels of PGE2 are found in various cancers, including hepatocellular carcinoma. The activation of the EP2 receptor pathway has been linked to increased Th17 cell differentiation in liver fibrosis models, suggesting that targeting this pathway could be beneficial in cancer therapy .
Research Findings
Recent investigations have provided insights into the mechanisms underlying the effects of this compound:
- Inflammation Modulation: Inflammatory stimuli such as lipopolysaccharides (LPS) significantly increase PGE2 levels in human bronchial epithelial cells. Inhibition studies using specific antagonists have shown a marked reduction in PGE2 release under inflammatory conditions .
- Cell Viability: Studies indicate that PGE2 can enhance the proliferation of certain cell types via EP receptor activation, further supporting its role in both normal physiological processes and pathological conditions like cancer .
Summary Table of Biological Activities
Biological Activity | Function | Context |
---|---|---|
Pro-inflammatory | Promotes Th17 differentiation | Autoimmune diseases |
Anti-inflammatory | Suppresses Th1 response | Chronic inflammation |
Tumor progression | Enhances COX-2 expression | Cancer |
Cell proliferation | Stimulates cell growth | Tissue repair and regeneration |
Q & A
Basic Research Questions
Q. What are the key methodological considerations for quantifying 20-Hydroxy-PGE2 in biological samples?
- Answer : Use liquid chromatography-tandem mass spectrometry (LC-MS/MS) to ensure specificity and sensitivity. Validate protocols by assessing recovery rates, matrix effects, and limits of detection/quantification. Include internal standards (e.g., deuterated analogs) to correct for variability . Document instrument parameters (e.g., ionization mode, collision energy) and calibration curves using certified reference materials. Maintain rigorous lab practices, including sample storage at -80°C to prevent degradation .
Q. How should researchers design a literature review to contextualize this compound’s role in eicosanoid signaling?
- Answer :
Database selection : Use SciFinder and Web of Science to retrieve peer-reviewed articles, prioritizing studies with mechanistic insights (e.g., receptor binding assays, knockout models).
Quality assessment : Exclude non-peer-reviewed sources; prioritize studies reporting raw data (e.g., concentration-response curves) over review articles.
Citation management : Use tools like Zotero to track sources and ensure proper attribution .
Q. What experimental controls are essential for in vitro studies investigating this compound’s effects on cellular pathways?
- Answer :
- Negative controls : Use vehicle-treated cells (e.g., ethanol or DMSO) to account for solvent effects.
- Positive controls : Include known agonists/antagonists of EP receptors (e.g., PGE2 or AH6809) to validate assay responsiveness.
- Technical replicates : Perform experiments in triplicate to assess intra-assay variability.
- Biological replicates : Use cells from ≥3 independent cultures to ensure generalizability .
Advanced Research Questions
Q. How can researchers resolve contradictions in reported EC₅₀ values for this compound across studies?
- Answer : Conduct a systematic review using the FINER framework (Feasible, Interesting, Novel, Ethical, Relevant) to identify confounding variables:
- Assay conditions : Compare buffer composition (e.g., calcium levels), temperature, and cell passage numbers.
- Data normalization : Assess whether studies used fold-change vs. absolute quantification.
- Statistical analysis : Apply meta-analysis tools (e.g., random-effects models) to quantify heterogeneity .
Q. What strategies optimize the extraction efficiency of this compound from complex matrices like plasma or tissue homogenates?
- Answer :
- Solvent selection : Test combinations of organic solvents (e.g., methanol:acetonitrile, 80:20) to maximize lipid recovery.
- Solid-phase extraction (SPE) : Use mixed-mode cartridges (e.g., C18 with ion-exchange resins) to reduce phospholipid interference.
- Method validation : Calculate extraction efficiency by spiking pre- and post-extraction samples with isotopically labeled standards .
Q. How should researchers integrate multi-omics data (e.g., lipidomics, transcriptomics) to elucidate this compound’s regulatory networks?
- Answer :
- Data alignment : Use bioinformatics pipelines (e.g., XCMS Online, MetaboAnalyst) to cross-reference lipidomic peaks with transcriptomic datasets (e.g., RNA-seq of COX-2/PGES pathways).
- Pathway enrichment : Apply tools like KEGG or Reactome to identify overrepresented pathways (e.g., arachidonic acid metabolism).
- Validation : Confirm computational predictions with functional assays (e.g., siRNA knockdown of candidate genes) .
Q. Data Analysis and Reproducibility
Q. What statistical methods are recommended for analyzing dose-response relationships of this compound?
- Answer :
- Non-linear regression : Fit data to sigmoidal curves (e.g., Hill equation) using software like GraphPad Prism.
- Error propagation : Calculate 95% confidence intervals for EC₅₀ values using bootstrapping.
- Outlier detection : Apply Grubbs’ test to exclude anomalous data points while documenting exclusion criteria .
Q. How can researchers ensure reproducibility when studying this compound’s effects across different cell lines?
- Answer :
- Standardization : Use authenticated cell lines (e.g., ATCC) and report mycoplasma testing results.
- Protocol sharing : Publish step-by-step methodologies, including incubation times and serum-free pretreatment durations.
- Data transparency : Deposit raw mass spectrometry files in public repositories (e.g., MetaboLights) .
Q. Ethical and Reporting Standards
Q. What ethical considerations apply when using human-derived samples for this compound research?
- Answer :
- Informed consent : Obtain IRB approval for collecting plasma/tissue samples, specifying intended use in lipidomic studies.
- Data anonymization : Remove identifiers from metadata to comply with GDPR/HIPAA regulations.
- Conflict of interest : Disclose funding sources (e.g., pharmaceutical sponsors) that might bias interpretation .
Q. How should researchers address variability in this compound measurements caused by circadian rhythms?
- Answer :
- Time-series sampling : Collect samples at consistent times (e.g., 8:00–10:00 AM) to minimize diurnal fluctuations.
- Covariate adjustment : Include time-of-collection as a fixed effect in linear mixed models.
- Reporting : State sampling protocols in methods sections to enhance cross-study comparability .
Propriétés
IUPAC Name |
(Z)-7-[(1R,2R,3R)-2-[(E,3S)-3,8-dihydroxyoct-1-enyl]-3-hydroxy-5-oxocyclopentyl]hept-5-enoic acid | |
---|---|---|
Source | PubChem | |
URL | https://pubchem.ncbi.nlm.nih.gov | |
Description | Data deposited in or computed by PubChem | |
InChI |
InChI=1S/C20H32O6/c21-13-7-3-4-8-15(22)11-12-17-16(18(23)14-19(17)24)9-5-1-2-6-10-20(25)26/h1,5,11-12,15-17,19,21-22,24H,2-4,6-10,13-14H2,(H,25,26)/b5-1-,12-11+/t15-,16+,17+,19+/m0/s1 | |
Source | PubChem | |
URL | https://pubchem.ncbi.nlm.nih.gov | |
Description | Data deposited in or computed by PubChem | |
InChI Key |
AZIGEYVZEVXWAD-NZGURKHLSA-N | |
Source | PubChem | |
URL | https://pubchem.ncbi.nlm.nih.gov | |
Description | Data deposited in or computed by PubChem | |
Canonical SMILES |
C1C(C(C(C1=O)CC=CCCCC(=O)O)C=CC(CCCCCO)O)O | |
Source | PubChem | |
URL | https://pubchem.ncbi.nlm.nih.gov | |
Description | Data deposited in or computed by PubChem | |
Isomeric SMILES |
C1[C@H]([C@@H]([C@H](C1=O)C/C=C\CCCC(=O)O)/C=C/[C@H](CCCCCO)O)O | |
Source | PubChem | |
URL | https://pubchem.ncbi.nlm.nih.gov | |
Description | Data deposited in or computed by PubChem | |
Molecular Formula |
C20H32O6 | |
Source | PubChem | |
URL | https://pubchem.ncbi.nlm.nih.gov | |
Description | Data deposited in or computed by PubChem | |
DSSTOX Substance ID |
DTXSID901316974 | |
Record name | 20-Hydroxy-PGE2 | |
Source | EPA DSSTox | |
URL | https://comptox.epa.gov/dashboard/DTXSID901316974 | |
Description | DSSTox provides a high quality public chemistry resource for supporting improved predictive toxicology. | |
Molecular Weight |
368.5 g/mol | |
Source | PubChem | |
URL | https://pubchem.ncbi.nlm.nih.gov | |
Description | Data deposited in or computed by PubChem | |
Physical Description |
Solid | |
Record name | 20-Hydroxy-PGE2 | |
Source | Human Metabolome Database (HMDB) | |
URL | http://www.hmdb.ca/metabolites/HMDB0003247 | |
Description | The Human Metabolome Database (HMDB) is a freely available electronic database containing detailed information about small molecule metabolites found in the human body. | |
Explanation | HMDB is offered to the public as a freely available resource. Use and re-distribution of the data, in whole or in part, for commercial purposes requires explicit permission of the authors and explicit acknowledgment of the source material (HMDB) and the original publication (see the HMDB citing page). We ask that users who download significant portions of the database cite the HMDB paper in any resulting publications. | |
CAS No. |
57930-95-7 | |
Record name | 20-Hydroxy-PGE2 | |
Source | CAS Common Chemistry | |
URL | https://commonchemistry.cas.org/detail?cas_rn=57930-95-7 | |
Description | CAS Common Chemistry is an open community resource for accessing chemical information. Nearly 500,000 chemical substances from CAS REGISTRY cover areas of community interest, including common and frequently regulated chemicals, and those relevant to high school and undergraduate chemistry classes. This chemical information, curated by our expert scientists, is provided in alignment with our mission as a division of the American Chemical Society. | |
Explanation | The data from CAS Common Chemistry is provided under a CC-BY-NC 4.0 license, unless otherwise stated. | |
Record name | 20-Hydroxyprostaglandin E2 | |
Source | ChemIDplus | |
URL | https://pubchem.ncbi.nlm.nih.gov/substance/?source=chemidplus&sourceid=0057930957 | |
Description | ChemIDplus is a free, web search system that provides access to the structure and nomenclature authority files used for the identification of chemical substances cited in National Library of Medicine (NLM) databases, including the TOXNET system. | |
Record name | 20-Hydroxy-PGE2 | |
Source | EPA DSSTox | |
URL | https://comptox.epa.gov/dashboard/DTXSID901316974 | |
Description | DSSTox provides a high quality public chemistry resource for supporting improved predictive toxicology. | |
Record name | 20-Hydroxyprostaglandin E2 | |
Source | FDA Global Substance Registration System (GSRS) | |
URL | https://gsrs.ncats.nih.gov/ginas/app/beta/substances/2DUQ67RPB3 | |
Description | The FDA Global Substance Registration System (GSRS) enables the efficient and accurate exchange of information on what substances are in regulated products. Instead of relying on names, which vary across regulatory domains, countries, and regions, the GSRS knowledge base makes it possible for substances to be defined by standardized, scientific descriptions. | |
Explanation | Unless otherwise noted, the contents of the FDA website (www.fda.gov), both text and graphics, are not copyrighted. They are in the public domain and may be republished, reprinted and otherwise used freely by anyone without the need to obtain permission from FDA. Credit to the U.S. Food and Drug Administration as the source is appreciated but not required. | |
Record name | 20-Hydroxy-PGE2 | |
Source | Human Metabolome Database (HMDB) | |
URL | http://www.hmdb.ca/metabolites/HMDB0003247 | |
Description | The Human Metabolome Database (HMDB) is a freely available electronic database containing detailed information about small molecule metabolites found in the human body. | |
Explanation | HMDB is offered to the public as a freely available resource. Use and re-distribution of the data, in whole or in part, for commercial purposes requires explicit permission of the authors and explicit acknowledgment of the source material (HMDB) and the original publication (see the HMDB citing page). We ask that users who download significant portions of the database cite the HMDB paper in any resulting publications. | |
Retrosynthesis Analysis
AI-Powered Synthesis Planning: Our tool employs the Template_relevance Pistachio, Template_relevance Bkms_metabolic, Template_relevance Pistachio_ringbreaker, Template_relevance Reaxys, Template_relevance Reaxys_biocatalysis model, leveraging a vast database of chemical reactions to predict feasible synthetic routes.
One-Step Synthesis Focus: Specifically designed for one-step synthesis, it provides concise and direct routes for your target compounds, streamlining the synthesis process.
Accurate Predictions: Utilizing the extensive PISTACHIO, BKMS_METABOLIC, PISTACHIO_RINGBREAKER, REAXYS, REAXYS_BIOCATALYSIS database, our tool offers high-accuracy predictions, reflecting the latest in chemical research and data.
Strategy Settings
Precursor scoring | Relevance Heuristic |
---|---|
Min. plausibility | 0.01 |
Model | Template_relevance |
Template Set | Pistachio/Bkms_metabolic/Pistachio_ringbreaker/Reaxys/Reaxys_biocatalysis |
Top-N result to add to graph | 6 |
Feasible Synthetic Routes
Avertissement et informations sur les produits de recherche in vitro
Veuillez noter que tous les articles et informations sur les produits présentés sur BenchChem sont destinés uniquement à des fins informatives. Les produits disponibles à l'achat sur BenchChem sont spécifiquement conçus pour des études in vitro, qui sont réalisées en dehors des organismes vivants. Les études in vitro, dérivées du terme latin "in verre", impliquent des expériences réalisées dans des environnements de laboratoire contrôlés à l'aide de cellules ou de tissus. Il est important de noter que ces produits ne sont pas classés comme médicaments et n'ont pas reçu l'approbation de la FDA pour la prévention, le traitement ou la guérison de toute condition médicale, affection ou maladie. Nous devons souligner que toute forme d'introduction corporelle de ces produits chez les humains ou les animaux est strictement interdite par la loi. Il est essentiel de respecter ces directives pour assurer la conformité aux normes légales et éthiques en matière de recherche et d'expérimentation.