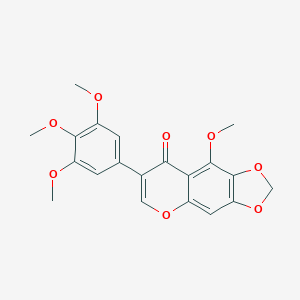
Irisflorentin
Vue d'ensemble
Description
L'irisflorentin est un flavonoïde naturel que l'on trouve principalement dans les plantes de la famille des Iridacées, en particulier dans l'herbe chinoise traditionnelle Belamcanda chinensis . Ce composé a fait l'objet de nombreuses recherches en raison de ses diverses activités pharmacologiques, notamment anti-inflammatoire, antitumorale, antidiabétique et sur le système nerveux central .
Méthodes De Préparation
Voies de synthèse et conditions de réaction : L'irisflorentin peut être isolé des racines de Belamcanda chinensis en utilisant diverses techniques d'extraction et de purification. La méthode la plus courante implique l'utilisation de solvants tels que le méthanol ou l'éthanol pour l'extraction, suivie de techniques chromatographiques pour la purification .
Méthodes de production industrielle : La production industrielle d'this compound implique généralement une extraction à grande échelle à partir de sources végétales. Des techniques de pointe telles que la chromatographie liquide haute performance (HPLC) sont utilisées pour assurer une pureté et un rendement élevés .
Analyse Des Réactions Chimiques
Phase I Metabolic Reactions
Irisflorentin undergoes oxidative metabolism primarily mediated by cytochrome P450 (CYP) enzymes in human liver microsomes (HLMs). Key findings include:
- Metabolite Formation : Incubation with HLMs generates 6,7-dihydroxy-5,3,4,5-tetramethoxyisoflavone via cleavage of the methylene acetal group (Fig. 1) . This metabolite exhibits enhanced pharmacological activity compared to the parent compound .
- Enzyme Specificity : CYP1A2 is the predominant enzyme responsible for this compound metabolism, with minor contributions from CYP2C9 and CYP3A4 .
Table 1: Kinetic Parameters of this compound Metabolism
Enzyme Kinetics and Cooperativity
This compound exhibits sigmoidal kinetics during CYP1A2-mediated metabolism, characterized by:
- Positive Cooperativity : The Eadie-Hofstee plot shows a convex "hooked" curve, indicating substrate activation at higher concentrations .
- Hill Equation Fit : Nonlinear regression analysis confirmed a Hill coefficient of 1.8 in HLMs and 1.5 in recombinant CYP1A2, suggesting multisite binding interactions .
Molecular Docking and Binding Mechanisms
Molecular simulations reveal critical interactions between this compound and CYP enzymes:
- CYP1A2 Binding : this compound binds to the substrate pocket via π-π stacking with Phe226 and hydrophobic interactions with Phe260 and Asn312 (Fig. 2) .
- Heme Proximity : The methylene acetal group aligns with the Fe center (4.0 Å), facilitating oxidative cleavage .
Figure 1: Proposed Metabolic Pathway of this compound
textThis compound → CYP1A2 → 6,7-dihydroxy-5,3,4,5-tetramethoxyisoflavone
Chemical Stability and Reactivity
- pH Sensitivity : this compound remains stable under neutral conditions but degrades in acidic environments (pH < 4) .
- Photodegradation : Prolonged UV exposure induces isomerization, reducing bioactivity .
Comparative Metabolism Across Species
Studies in rat liver microsomes show similar metabolic profiles to humans, with a Hill coefficient of 1.48 for CYP1A2, supporting cross-species extrapolation .
Applications De Recherche Scientifique
Medicinal Properties
Irisflorentin has been recognized for its potential therapeutic effects against several diseases, including:
- Inflammatory Disorders : this compound exhibits anti-inflammatory properties by modulating dendritic cell responses and reducing pro-inflammatory cytokine production. In vitro studies have shown that it significantly inhibits the maturation of dendritic cells stimulated by lipopolysaccharides (LPS), leading to decreased production of tumor necrosis factor-α (TNF-α), interleukin-6 (IL-6), and interleukin-12p70 (IL-12) .
- Cancer Treatment : Research indicates that this compound may have anti-cancer effects, particularly in breast cancer. It has been studied for its ability to inhibit the breast cancer resistance protein (BCRP), which is associated with drug resistance in cancer therapies . Additionally, it has shown promise in attenuating dopaminergic neuron degeneration linked to Parkinson's disease, suggesting a protective role against neurodegenerative disorders .
- Diabetes Management : this compound has demonstrated inhibitory effects on α-glucosidase, an enzyme involved in carbohydrate digestion, indicating its potential use as an anti-diabetic agent .
Pharmacological Activity
The pharmacological activities of this compound can be summarized as follows:
Analytical Methods
Various analytical techniques have been employed to study this compound's properties:
- High-Performance Liquid Chromatography (HPLC) : This method is used for quantifying this compound in biological samples, ensuring accurate measurement of its concentration during pharmacological studies .
- Mass Spectrometry : Coupled with HPLC, mass spectrometry provides detailed insights into the metabolic pathways and pharmacokinetics of this compound, enhancing our understanding of its biological effectiveness .
Case Studies
Several case studies illustrate the applications of this compound:
- Study on Allergic Responses : A study demonstrated that this compound treatment reduced allergic contact hypersensitivity responses in mice by modulating dendritic cell activity and cytokine production, highlighting its potential as an immunotherapeutic agent .
- Neuroprotective Effects : Another investigation focused on the neuroprotective effects of this compound against oxidative stress-induced dopaminergic neuron degeneration. The results indicated that it improved α-synuclein accumulation and offered protection against neurodegeneration induced by 6-hydroxydopamine (6-OHDA) .
Mécanisme D'action
Irisflorentin exerts its effects through various molecular targets and pathways:
Anti-inflammatory: Inhibits the production of pro-inflammatory cytokines and enzymes such as inducible nitric oxide synthase (iNOS).
Antitumor: Induces apoptosis in cancer cells by modulating signaling pathways such as the MAPK pathway.
Antidiabetic: Inhibits α-glucosidase, thereby reducing blood glucose levels .
Comparaison Avec Des Composés Similaires
- Rhapontigenin
- Trans-resveratrol
- 5,7,4’-Trihydroxy-6,3’,5’-trimethoxy-isoflavone
- 6-Hydroxybiochannin A
- Iridin S
- Pinoresinol
- Isoiridogermanal
- Iristectorene B
Uniqueness: Irisflorentin is unique due to its specific substitution pattern on the flavonoid skeleton, which imparts distinct pharmacological properties. Its high receptor-binding capacity and diverse bioactivities make it a valuable compound for various scientific and medical applications .
Activité Biologique
Irisflorentin, a naturally occurring isoflavone predominantly found in Belamcanda chinensis (also known as Iris domestica), has garnered attention for its diverse biological activities. This article explores the compound's anti-inflammatory, neuroprotective, and anticancer properties, supported by various studies and case reports.
Overview of this compound
This compound is recognized for its pharmacological potential, particularly in traditional medicine. It has been utilized for treating various ailments, including inflammatory disorders, respiratory issues, and even as an antidote in traditional Chinese medicine. Its bioactive properties are attributed to its flavonoid structure, which is known to exert multiple health benefits.
Anti-Inflammatory Activity
A significant body of research highlights the anti-inflammatory effects of this compound. In a study involving lipopolysaccharide (LPS)-stimulated RAW 264.7 macrophages, this compound was shown to inhibit the production of pro-inflammatory cytokines such as TNF-α, IL-1β, and IL-6. The mechanism involves the downregulation of inducible nitric oxide synthase (iNOS) and the modulation of signaling pathways, specifically through ERK1/2 and p38 MAPK rather than the NF-κB pathway .
Table 1: Summary of Anti-Inflammatory Effects
Study | Cell Type | Treatment | Key Findings |
---|---|---|---|
RAW 264.7 | LPS + this compound | Reduced iNOS expression and NO production; inhibited TNF-α, IL-1β, IL-6 | |
HMC3 Cells | This compound | Blocked NLRP3 inflammasome activation |
Neuroprotective Effects
This compound shows promise in neuroprotection, particularly concerning neurodegenerative diseases such as Parkinson's disease (PD). In a Caenorhabditis elegans model of PD, this compound prevented α-synuclein accumulation and improved dopaminergic neuron survival. The compound appears to enhance proteasomal activity while inhibiting apoptotic pathways .
Table 2: Neuroprotective Effects in C. elegans Models
Study | Model Organism | Treatment | Outcome |
---|---|---|---|
C. elegans | This compound | Reduced α-synuclein accumulation; improved neuron survival and lifespan |
Anticancer Properties
Research indicates that this compound possesses anticancer properties. It has been shown to inhibit cancer cell proliferation and induce apoptosis in various cancer cell lines. The underlying mechanisms involve modulation of cell cycle regulators and apoptotic pathways .
Table 3: Anticancer Activity Overview
Study | Cell Line | Treatment | Effect Observed |
---|---|---|---|
Various Cancer Lines | This compound | Inhibition of cell growth; induction of apoptosis |
Case Studies and Clinical Insights
While most findings are based on in vitro studies or animal models, there is a growing interest in clinical applications. For instance, this compound's role as an immunotherapeutic adjuvant has been explored, suggesting its potential to enhance vaccine efficacy or other therapeutic interventions .
Propriétés
IUPAC Name |
9-methoxy-7-(3,4,5-trimethoxyphenyl)-[1,3]dioxolo[4,5-g]chromen-8-one | |
---|---|---|
Source | PubChem | |
URL | https://pubchem.ncbi.nlm.nih.gov | |
Description | Data deposited in or computed by PubChem | |
InChI |
InChI=1S/C20H18O8/c1-22-13-5-10(6-14(23-2)18(13)24-3)11-8-26-12-7-15-19(28-9-27-15)20(25-4)16(12)17(11)21/h5-8H,9H2,1-4H3 | |
Source | PubChem | |
URL | https://pubchem.ncbi.nlm.nih.gov | |
Description | Data deposited in or computed by PubChem | |
InChI Key |
RISXUTCDCPHJFQ-UHFFFAOYSA-N | |
Source | PubChem | |
URL | https://pubchem.ncbi.nlm.nih.gov | |
Description | Data deposited in or computed by PubChem | |
Canonical SMILES |
COC1=CC(=CC(=C1OC)OC)C2=COC3=CC4=C(C(=C3C2=O)OC)OCO4 | |
Source | PubChem | |
URL | https://pubchem.ncbi.nlm.nih.gov | |
Description | Data deposited in or computed by PubChem | |
Molecular Formula |
C20H18O8 | |
Source | PubChem | |
URL | https://pubchem.ncbi.nlm.nih.gov | |
Description | Data deposited in or computed by PubChem | |
DSSTOX Substance ID |
DTXSID60194575 | |
Record name | Irisflorentin | |
Source | EPA DSSTox | |
URL | https://comptox.epa.gov/dashboard/DTXSID60194575 | |
Description | DSSTox provides a high quality public chemistry resource for supporting improved predictive toxicology. | |
Molecular Weight |
386.4 g/mol | |
Source | PubChem | |
URL | https://pubchem.ncbi.nlm.nih.gov | |
Description | Data deposited in or computed by PubChem | |
CAS No. |
41743-73-1 | |
Record name | Irisflorentin | |
Source | CAS Common Chemistry | |
URL | https://commonchemistry.cas.org/detail?cas_rn=41743-73-1 | |
Description | CAS Common Chemistry is an open community resource for accessing chemical information. Nearly 500,000 chemical substances from CAS REGISTRY cover areas of community interest, including common and frequently regulated chemicals, and those relevant to high school and undergraduate chemistry classes. This chemical information, curated by our expert scientists, is provided in alignment with our mission as a division of the American Chemical Society. | |
Explanation | The data from CAS Common Chemistry is provided under a CC-BY-NC 4.0 license, unless otherwise stated. | |
Record name | Irisflorentin | |
Source | ChemIDplus | |
URL | https://pubchem.ncbi.nlm.nih.gov/substance/?source=chemidplus&sourceid=0041743731 | |
Description | ChemIDplus is a free, web search system that provides access to the structure and nomenclature authority files used for the identification of chemical substances cited in National Library of Medicine (NLM) databases, including the TOXNET system. | |
Record name | Irisflorentin | |
Source | EPA DSSTox | |
URL | https://comptox.epa.gov/dashboard/DTXSID60194575 | |
Description | DSSTox provides a high quality public chemistry resource for supporting improved predictive toxicology. | |
Retrosynthesis Analysis
AI-Powered Synthesis Planning: Our tool employs the Template_relevance Pistachio, Template_relevance Bkms_metabolic, Template_relevance Pistachio_ringbreaker, Template_relevance Reaxys, Template_relevance Reaxys_biocatalysis model, leveraging a vast database of chemical reactions to predict feasible synthetic routes.
One-Step Synthesis Focus: Specifically designed for one-step synthesis, it provides concise and direct routes for your target compounds, streamlining the synthesis process.
Accurate Predictions: Utilizing the extensive PISTACHIO, BKMS_METABOLIC, PISTACHIO_RINGBREAKER, REAXYS, REAXYS_BIOCATALYSIS database, our tool offers high-accuracy predictions, reflecting the latest in chemical research and data.
Strategy Settings
Precursor scoring | Relevance Heuristic |
---|---|
Min. plausibility | 0.01 |
Model | Template_relevance |
Template Set | Pistachio/Bkms_metabolic/Pistachio_ringbreaker/Reaxys/Reaxys_biocatalysis |
Top-N result to add to graph | 6 |
Feasible Synthetic Routes
Avertissement et informations sur les produits de recherche in vitro
Veuillez noter que tous les articles et informations sur les produits présentés sur BenchChem sont destinés uniquement à des fins informatives. Les produits disponibles à l'achat sur BenchChem sont spécifiquement conçus pour des études in vitro, qui sont réalisées en dehors des organismes vivants. Les études in vitro, dérivées du terme latin "in verre", impliquent des expériences réalisées dans des environnements de laboratoire contrôlés à l'aide de cellules ou de tissus. Il est important de noter que ces produits ne sont pas classés comme médicaments et n'ont pas reçu l'approbation de la FDA pour la prévention, le traitement ou la guérison de toute condition médicale, affection ou maladie. Nous devons souligner que toute forme d'introduction corporelle de ces produits chez les humains ou les animaux est strictement interdite par la loi. Il est essentiel de respecter ces directives pour assurer la conformité aux normes légales et éthiques en matière de recherche et d'expérimentation.