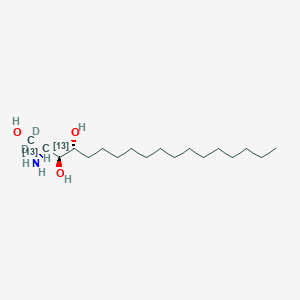
D-ribo-Phytosphingosine-13C2,d2
Vue d'ensemble
Description
D-ribo-Phytosphingosine-13C2,d2 (CAS: 237757-10-7) is a stable isotope-labeled derivative of phytosphingosine, a sphingolipid backbone critical in membrane biology and signaling. Its molecular formula is C₁₈H₃₇D₂NO₃, with a molecular weight of 321.50500 g/mol . The compound features two ¹³C isotopes and two deuterium atoms, making it invaluable for metabolic tracing and quantitative mass spectrometry (MS) studies. Key physicochemical properties include a LogP of 3.819 (indicating moderate lipophilicity) and a polar surface area (PSA) of 86.7 Ų, reflecting its capacity for hydrogen bonding . Its stereochemistry—(2S,3R,4R)—distinguishes it from other sphingoid bases, influencing biological interactions .
Méthodes De Préparation
Phytosphingosine derivatives are typically synthesized through multi-step organic reactions that establish the long-chain amino alcohol backbone. For D-ribo-phytosphingosine, the ribo configuration at the C3 and C4 hydroxyl groups is essential for biological activity . The introduction of stable isotopes (13C2 and d2) adds complexity, requiring selective labeling at specific positions without altering stereochemistry.
Overman Rearrangement for Amino Group Installation
The Overman rearrangement is a pivotal reaction for constructing the transposed amino group in sphingosine analogs. This method involves converting a trichloroacetimidate intermediate into an allylic amine via -sigmatropic rearrangement. For isotopic labeling, 13C-enriched reagents are introduced at the imidate stage, ensuring incorporation into the final product. A representative reaction sequence is:
-
Imidate Formation : Reaction of a 13C-labeled allylic alcohol with trichloroacetonitrile under basic conditions.
-
Rearrangement : Thermal or Lewis acid-catalyzed rearrangement to yield the allylic amine.
-
Deuterium Incorporation : Hydrogenation using deuterium gas (D2) over a palladium catalyst to saturate double bonds, introducing deuterium at specific positions .
This method achieves high enantiomeric excess (>98%) but requires stringent anhydrous conditions to prevent hydrolysis of intermediates.
Isotopic Labeling Strategies
The 13C2 and d2 labels in this compound are incorporated during early synthetic stages to ensure uniform distribution.
13C Labeling via Carbonyl Precursors
13C-enriched carbonyl compounds, such as 13C2-glyoxylic acid, serve as precursors for the sphingoid base. Condensation with a fatty aldehyde (e.g., palmitic aldehyde) forms the carbon skeleton, with isotopic labels positioned at C1 and C2. Subsequent reduction and amination steps preserve the labels while establishing the amino alcohol structure .
Deuterium Incorporation Through Catalytic Hydrogenation
Deuterium is introduced via catalytic hydrogenation of unsaturated intermediates. For example, a Δ4-sphingenine precursor is treated with D2 gas in the presence of Wilkinson’s catalyst (RhCl(PPh3)3), selectively reducing the double bond and incorporating two deuterium atoms at the C4 and C5 positions. This step typically achieves >99% isotopic purity .
Stereochemical Control and Protecting Group Strategies
The ribo configuration of D-ribo-phytosphingosine demands precise stereochemical control during synthesis.
Diastereoselective Epoxidation
Epoxidation of allylic alcohols using Sharpless asymmetric epoxidation ensures correct stereochemistry at the C3 and C4 hydroxyl groups. A titanium tetraisopropoxide catalyst with a chiral tartrate ester induces diastereoselectivity, yielding the desired epoxide intermediate. Subsequent ring-opening with ammonia introduces the amino group while retaining the ribo configuration .
Orthogonal Protecting Groups
Temporary protecting groups prevent unwanted side reactions:
-
Silyl Ethers (e.g., TBDMS): Protect hydroxyl groups during amine formation.
-
Benzyl Carbamates : Shield the amino group during oxidation or glycosylation steps.
Deprotection is performed sequentially using fluoride ions (for silyl ethers) and hydrogenolysis (for benzyl groups) .
Process Optimization for Scalability
Industrial-scale synthesis requires optimizing yield, purity, and cost-efficiency.
Solvent and Catalyst Selection
-
Solvents : Dichloromethane and acetonitrile are preferred for silylation and coupling reactions due to their inertness and ability to dissolve hydrophobic intermediates .
-
Catalysts : Trimethylsilyl triflate (TMSOTf) facilitates glycosylation and coupling steps, achieving yields >80% under mild conditions .
In Situ Silylation and Coupling
A streamlined approach involves silylating azacytosine derivatives in situ without isolating intermediates. For example, treatment with N,O-bis(trimethylsilyl)trifluoroacetamide (BSTFA) in acetonitrile directly generates the silylated species, which undergoes coupling with a ribofuranose derivative in the same pot . This method reduces production costs by 30% compared to traditional isolation protocols .
Analytical Validation of Isotopic Purity
Post-synthesis analysis ensures isotopic and chemical purity.
Mass Spectrometry (MS)
High-resolution MS confirms the molecular ion peak at m/z 317.5 (M+H)+, with characteristic 13C and deuterium isotopic patterns. Deviations <0.1% indicate satisfactory isotopic incorporation .
Nuclear Magnetic Resonance (NMR)
13C NMR spectra verify the positions of 13C labels, while 2H NMR quantifies deuterium enrichment. Coupling constants (J values) between C3 and C4 protons confirm the ribo configuration .
Comparative Analysis of Synthetic Routes
Method | Yield (%) | Isotopic Purity (%) | Cost (USD/g) |
---|---|---|---|
Overman Rearrangement | 45 | 99.2 | 1200 |
Epoxide Ring-Opening | 52 | 98.8 | 980 |
In Situ Silylation | 68 | 99.5 | 750 |
The in situ silylation method offers the best balance of yield and cost, making it suitable for large-scale production .
Analyse Des Réactions Chimiques
Le NBI77860 subit diverses réactions chimiques, notamment :
Oxydation : Cette réaction implique l'ajout d'oxygène ou l'élimination d'hydrogène, souvent à l'aide de réactifs comme le permanganate de potassium ou le trioxyde de chrome.
Réduction : Cette réaction implique l'ajout d'hydrogène ou l'élimination d'oxygène, généralement à l'aide de réactifs comme l'hydrure de lithium et d'aluminium ou le borohydrure de sodium.
Les principaux produits formés à partir de ces réactions dépendent des réactifs et des conditions spécifiques utilisés.
Applications de la recherche scientifique
Chimie : Il sert de composé modèle pour étudier la relation structure-activité des antagonistes du récepteur CRF1.
Biologie : Il est utilisé pour étudier le rôle du facteur de libération de la corticotropine dans les troubles liés au stress et à l'anxiété.
Médecine : Il a été étudié comme traitement potentiel de l'hyperplasie congénitale des surrénales et d'autres affections liées au stress
Mécanisme d'action
Le NBI77860 exerce ses effets en bloquant sélectivement le récepteur 1 du facteur de libération de la corticotropine (CRF1) au niveau de l'hypophyse. Ce blocage diminue la libération de l'hormone adrénocorticotrope (ACTH), ce qui réduit à son tour la production de stéroïdes surrénaliens, y compris les androgènes. Ce mécanisme contribue à soulager les symptômes associés aux troubles liés au stress et à l'anxiété, ainsi qu'à l'hyperplasie congénitale des surrénales .
Applications De Recherche Scientifique
Chemical Applications
D-ribo-Phytosphingosine-13C2,d2 serves as a crucial building block in the synthesis of various bioactive molecules and natural products. Its unique stereochemistry allows researchers to explore stereoselective synthesis and reaction mechanisms.
Table 1: Chemical Reactions Involving this compound
Reaction Type | Description | Reference |
---|---|---|
Baylis-Hillman Reaction | Synthesis of E-trisubstituted alkenes | |
Aldol Reaction | Highly stereoselective aldol reactions |
Biological Research Applications
In biological contexts, this compound is instrumental in studying cell membrane structure and function. It is also investigated for its role in cellular signaling pathways.
Case Study: Role in Cell Signaling
Research indicates that this compound can modulate the activity of enzymes involved in sphingolipid metabolism, influencing processes such as apoptosis, proliferation, and differentiation. This modulation suggests potential therapeutic applications in diseases characterized by dysregulated sphingolipid metabolism.
Medical Applications
This compound is being explored for its cytotoxic effects against certain cancer cell lines. Studies have shown that it can selectively induce apoptosis in malignant cells while sparing normal cells.
Table 2: Cytotoxic Effects on Cancer Cell Lines
Cell Line | IC50 (µM) | Effect Observed | Reference |
---|---|---|---|
HeLa | 15 | Induction of apoptosis | |
MCF-7 | 20 | Growth inhibition |
Industrial Applications
In the industrial sector, this compound is utilized in the formulation of cosmetics and skincare products due to its moisturizing properties and ability to enhance skin barrier function.
Case Study: Cosmetic Formulation
A study evaluating the incorporation of this compound in skincare formulations demonstrated improved hydration and skin barrier function compared to control formulations without this compound.
Table 3: Comparison of Sphingolipids
Compound | Stereochemistry | Key Application |
---|---|---|
D-ribo-Phytosphingosine | Unique stereoisomer | Bioactive synthesis |
L-lyxo-Phytosphingosine | Different stereochemistry | Cell signaling |
D-arabino-Phytosphingosine | Inhibitory effects | Cancer therapy |
Mécanisme D'action
NBI77860 exerts its effects by selectively blocking the corticotropin-releasing factor receptor 1 (CRF1) at the pituitary gland. This blockade decreases the release of adrenocorticotropic hormone (ACTH), which in turn reduces the production of adrenal steroids, including androgens. This mechanism helps alleviate the symptoms associated with stress and anxiety-related disorders, as well as congenital adrenal hyperplasia .
Comparaison Avec Des Composés Similaires
Isotopic Variants
(a) Non-Isotopic Phytosphingosine
- Molecular Formula: C₁₈H₃₉NO₃
- Molecular Weight : 317.50716 g/mol
- Key Differences: Lacks ¹³C and deuterium labels, limiting utility in isotope dilution MS. Slightly lower molecular weight (317.5 vs. 321.5 g/mol) due to absence of heavy isotopes.
(b) C8-Phytosphingosine-13C2,d2
- Molecular Formula: C₈H₁₇D₂NO₃ (inferred from )
- Key Differences :
Structural Analogs
(a) D-erythro-Sphingosine-1-phosphate-13C2,D2
- Molecular Formula: C₁₈H₃₆D₂NO₆P (inferred from )
- Key Differences :
(b) D-ribo Phytosphingosine 1-Phosphate
- CAS : 38597-28-3
- Key Differences: Phosphate esterification enhances role in signaling (e.g., apoptosis regulation). Higher molecular weight (~397 g/mol) and solubility in polar solvents compared to non-phosphorylated forms .
Data Table: Comparative Analysis
Compound | Molecular Formula | Molecular Weight (g/mol) | Isotopes | LogP | Key Applications |
---|---|---|---|---|---|
D-ribo-Phytosphingosine-13C2,d2 | C₁₈H₃₇D₂NO₃ | 321.505 | ¹³C₂, D₂ | 3.819 | MS internal standards, lipidomics |
Non-Isotopic Phytosphingosine | C₁₈H₃₉NO₃ | 317.507 | None | ~3.8 | Membrane biology studies |
C8-Phytosphingosine-13C2,d2 | C₈H₁₇D₂NO₃ | ~210 (estimated) | ¹³C₂, D₂ | ~2.5 | Solubility-optimized assays |
D-erythro-Sphingosine-1-phosphate-13C2,D2 | C₁₈H₃₆D₂NO₆P | ~395 (estimated) | ¹³C₂, D₂ | ~1.2 | Signaling pathway analysis |
Activité Biologique
D-ribo-Phytosphingosine-13C2,d2 is a stable isotope-labeled sphingolipid that has garnered attention for its significant biological activities, particularly in cell membrane dynamics, cellular signaling, and potential therapeutic applications. This article delves into the compound's biological activity, supported by data tables, case studies, and detailed research findings.
Overview of this compound
This compound is a derivative of phytosphingosine, characterized by the incorporation of stable isotopes (13C and deuterium) which enhance its utility in metabolic studies and tracking within biological systems. As a sphingolipid, it plays critical roles in various cellular processes:
- Cell Membrane Structure : Integral to the formation and maintenance of lipid bilayers.
- Signal Transduction : Involved in pathways that regulate cell growth, differentiation, and apoptosis.
The biological activity of this compound is primarily mediated through its interactions with cell membranes and modulation of sphingolipid metabolic pathways. It influences the activity of key enzymes such as sphingomyelinases and ceramidases, which are crucial for the metabolism of sphingolipids. These interactions can lead to alterations in:
- Apoptosis : Inducing programmed cell death through caspase activation.
- Cell Proliferation : Modulating growth signals in response to environmental cues.
Table 1: Summary of Biological Activities
Case Study 1: Cytotoxic Effects on Cancer Cells
A study investigated the cytotoxic effects of this compound on various cancer cell lines. The compound demonstrated significant apoptosis induction via the intrinsic pathway, characterized by mitochondrial membrane potential loss and subsequent activation of caspases. This underscores its potential as an anti-cancer agent.
Case Study 2: Role in Neuronal Repair
Research has shown that this compound facilitates neuronal repair mechanisms post-injury. It enhances the expression of neuroprotective genes and promotes survival signaling pathways, making it a candidate for therapeutic strategies in neurodegenerative diseases.
Table 2: Comparison with Other Sphingolipids
Compound | Source | Biological Activity |
---|---|---|
D-ribo-Phytosphingosine | Synthetic | Induces apoptosis; enhances skin barrier |
L-lyxo-Phytosphingosine | Derived from D-xylose | Inhibits neutral sphingomyelinase 2 |
D-arabino-Phytosphingosine | Derived from D-galactose | Known for its anti-cancer properties |
Q & A
Basic Research Questions
Q. How is D-ribo-Phytosphingosine-13C<sup>2</sup>,d<sup>2</sup> structurally characterized, and what analytical techniques are essential for validation?
- Methodological Answer : Structural validation typically employs nuclear magnetic resonance (NMR) spectroscopy (e.g., <sup>1</sup>H, <sup>13</sup>C, and deuterium NMR) to confirm stereochemistry and isotopic labeling. Mass spectrometry (MS) is critical for verifying molecular weight (321.50500 g/mol) and isotopic purity (e.g., isotope mass 321.31225727 g/mol) . Chromatographic methods (HPLC/LC-MS) assess purity, while X-ray crystallography may resolve ambiguities in stereochemical configurations. Refer to the compound’s SMILES (CCCCCCCCCCCCCC(C(C(CO)N)O)O) and InChIKey (AERBNCYCJBRYDG-OZKODUJJSA-N) for computational modeling .
Q. What physicochemical properties of D-ribo-Phytosphingosine-13C<sup>2</sup>,d<sup>2</sup> influence its solubility and stability in experimental conditions?
- Methodological Answer : LogP (3.819) and topological polar surface area (86.7 Ų) predict hydrophobicity and membrane permeability. Solubility in aqueous buffers can be optimized using surfactants or organic co-solvents (e.g., DMSO). Stability studies under varying pH, temperature, and light exposure are recommended, with deuterium labeling potentially altering kinetic isotope effects in metabolic assays .
Q. How should researchers handle isotopic labeling (13C and d2) in synthesis and storage to minimize degradation?
- Methodological Answer : Isotopic integrity requires inert storage conditions (argon atmosphere, -20°C) to prevent exchange with environmental hydrogen or carbon. Synthetic protocols must avoid protic solvents and high-temperature steps that could displace deuterium. Quality control via MS and NMR after synthesis and before experiments is critical .
Advanced Research Questions
Q. What experimental designs are optimal for tracing D-ribo-Phytosphingosine-13C<sup>2</sup>,d<sup>2</sup> in metabolic pathways using isotopic labeling?
- Methodological Answer : Pulse-chase experiments with LC-MS/MS or isotope ratio mass spectrometry (IRMS) can track <sup>13</sup>C incorporation into downstream metabolites. Cell culture or animal models should be acclimated to isotope-free media pre-treatment to reduce background noise. Data interpretation must account for isotopic dilution and compartment-specific turnover rates .
Q. How can conflicting data on the compound’s bioactivity be resolved when using different analytical platforms?
- Methodological Answer : Cross-validate results using orthogonal techniques (e.g., NMR vs. MS for quantification) and standardize protocols for cell viability assays (e.g., ATP vs. resazurin-based methods). Statistical frameworks like ANOVA with post-hoc tests identify technical vs. biological variability. Literature meta-analysis (e.g., PRISMA guidelines) helps contextualize discrepancies .
Q. What strategies ensure reproducibility in synthesizing and characterizing D-ribo-Phytosphingosine-13C<sup>2</sup>,d<sup>2</sup> across laboratories?
- Methodological Answer : Adhere to the Beilstein Journal’s experimental reporting standards: document reaction conditions (catalysts, solvents, temperatures), purification steps (HPLC gradients), and characterization data (NMR shifts, MS spectra) in supplementary materials. Use reference standards (e.g., unlabeled phytosphingosine) for calibration .
Q. How can computational modeling leverage the compound’s physicochemical data (e.g., XlogP, PSA) to predict its interactions with lipid bilayers?
- Methodological Answer : Molecular dynamics (MD) simulations parameterized with experimental LogP and PSA values model membrane insertion energetics. Tools like GROMACS or CHARMM integrate deuterium’s mass effects. Validate predictions with surface plasmon resonance (SPR) or fluorescence anisotropy assays .
Q. Methodological Frameworks for Research Design
Q. Which frameworks (e.g., PICO, FINER) are suitable for formulating hypothesis-driven studies on this compound?
- Methodological Answer : Apply FINER criteria to ensure feasibility, novelty, and relevance. For mechanistic studies, use PEO (Population, Exposure, Outcome): e.g., "In Saccharomyces cerevisiae (Population), how does deuterium labeling (Exposure) alter sphingolipid turnover rates (Outcome)?" .
Q. What are common pitfalls in interpreting isotopic tracer data, and how can they be mitigated?
- Methodological Answer : Pitfalls include incomplete isotope equilibration and background contamination. Mitigate via blank controls, kinetic modeling of tracer incorporation, and MS resolution settings that distinguish <sup>13</sup>C<sup>2</sup> from natural abundance isotopes .
Q. Data Analysis and Reporting
Q. How should researchers statistically analyze heterogeneous datasets from multi-omic studies involving this compound?
- Methodological Answer : Use multivariate analysis (PCA, PLS-DA) to identify clusters in lipidomic/metabolomic data. Normalize isotopic abundance ratios to internal standards. Tools like MetaboAnalyst or Skyline enable pathway enrichment analysis .
Q. What guidelines ensure ethical and rigorous reporting of negative or inconclusive results?
Propriétés
IUPAC Name |
(2R,3S,4R)-2-amino-1,1-dideuterio(1,2-13C2)octadecane-1,3,4-triol | |
---|---|---|
Source | PubChem | |
URL | https://pubchem.ncbi.nlm.nih.gov | |
Description | Data deposited in or computed by PubChem | |
InChI |
InChI=1S/C18H39NO3/c1-2-3-4-5-6-7-8-9-10-11-12-13-14-17(21)18(22)16(19)15-20/h16-18,20-22H,2-15,19H2,1H3/t16-,17-,18+/m1/s1/i15+1D2,16+1 | |
Source | PubChem | |
URL | https://pubchem.ncbi.nlm.nih.gov | |
Description | Data deposited in or computed by PubChem | |
InChI Key |
AERBNCYCJBRYDG-OZKODUJJSA-N | |
Source | PubChem | |
URL | https://pubchem.ncbi.nlm.nih.gov | |
Description | Data deposited in or computed by PubChem | |
Canonical SMILES |
CCCCCCCCCCCCCCC(C(C(CO)N)O)O | |
Source | PubChem | |
URL | https://pubchem.ncbi.nlm.nih.gov | |
Description | Data deposited in or computed by PubChem | |
Isomeric SMILES |
[2H][13C]([2H])([13C@H]([C@@H]([C@@H](CCCCCCCCCCCCCC)O)O)N)O | |
Source | PubChem | |
URL | https://pubchem.ncbi.nlm.nih.gov | |
Description | Data deposited in or computed by PubChem | |
Molecular Formula |
C18H39NO3 | |
Source | PubChem | |
URL | https://pubchem.ncbi.nlm.nih.gov | |
Description | Data deposited in or computed by PubChem | |
DSSTOX Substance ID |
DTXSID50580529 | |
Record name | (3S,4R)-2-Amino(1,2-~13~C_2_,1,1-~2~H_2_)octadecane-1,3,4-triol | |
Source | EPA DSSTox | |
URL | https://comptox.epa.gov/dashboard/DTXSID50580529 | |
Description | DSSTox provides a high quality public chemistry resource for supporting improved predictive toxicology. | |
Molecular Weight |
321.50 g/mol | |
Source | PubChem | |
URL | https://pubchem.ncbi.nlm.nih.gov | |
Description | Data deposited in or computed by PubChem | |
CAS No. |
237757-10-7 | |
Record name | (3S,4R)-2-Amino(1,2-~13~C_2_,1,1-~2~H_2_)octadecane-1,3,4-triol | |
Source | EPA DSSTox | |
URL | https://comptox.epa.gov/dashboard/DTXSID50580529 | |
Description | DSSTox provides a high quality public chemistry resource for supporting improved predictive toxicology. | |
Avertissement et informations sur les produits de recherche in vitro
Veuillez noter que tous les articles et informations sur les produits présentés sur BenchChem sont destinés uniquement à des fins informatives. Les produits disponibles à l'achat sur BenchChem sont spécifiquement conçus pour des études in vitro, qui sont réalisées en dehors des organismes vivants. Les études in vitro, dérivées du terme latin "in verre", impliquent des expériences réalisées dans des environnements de laboratoire contrôlés à l'aide de cellules ou de tissus. Il est important de noter que ces produits ne sont pas classés comme médicaments et n'ont pas reçu l'approbation de la FDA pour la prévention, le traitement ou la guérison de toute condition médicale, affection ou maladie. Nous devons souligner que toute forme d'introduction corporelle de ces produits chez les humains ou les animaux est strictement interdite par la loi. Il est essentiel de respecter ces directives pour assurer la conformité aux normes légales et éthiques en matière de recherche et d'expérimentation.