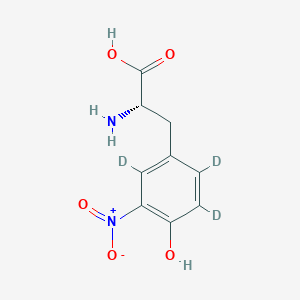
3-Nitro-L-tyrosine-d3
Vue d'ensemble
Description
(2S)-2-Amino-3-(2,3,6-trideuterio-4-hydroxy-5-nitrophenyl)propanoic acid: is a deuterated analog of a phenylalanine derivative. This compound is characterized by the presence of three deuterium atoms, a hydroxyl group, and a nitro group on the phenyl ring. The deuterium atoms replace hydrogen atoms, which can significantly alter the compound’s physical and chemical properties, making it valuable for various scientific research applications.
Applications De Recherche Scientifique
Chemistry:
- Used as a tracer in reaction mechanisms to study isotope effects.
- Employed in the synthesis of deuterated pharmaceuticals to improve metabolic stability.
Biology:
- Utilized in metabolic studies to trace biochemical pathways.
- Helps in understanding enzyme-substrate interactions by providing isotopic labeling.
Medicine:
- Deuterated analogs are used in drug development to enhance the pharmacokinetic properties of drugs.
- Investigated for potential therapeutic applications due to altered metabolic profiles.
Industry:
- Applied in the production of deuterated solvents and reagents for NMR spectroscopy.
- Used in the development of stable isotopic standards for mass spectrometry.
Mécanisme D'action
Target of Action
The primary target of 3-Nitro-L-tyrosine-d3, also known as (2S)-2-Amino-3-(2,3,6-trideuterio-4-hydroxy-5-nitrophenyl)propanoic acid, is Glutathione reductase, a mitochondrial enzyme . This enzyme plays a crucial role in maintaining the reduced form of glutathione, an important antioxidant in cells.
Mode of Action
This compound is formed when reactive nitrogen species, such as peroxynitrite (ONOO−) and nitryl chloride (NO2Cl), react with the aromatic ring of tyrosine in soluble amino acids and proteins . The compound’s interaction with its targets leads to the nitration of tyrosine residues, which can alter protein function and signaling pathways .
Biochemical Pathways
The formation of this compound is part of a broader biochemical pathway involving the reaction of nitric oxide (·NO) with oxygen in various forms in aqueous media . This reaction leads to the formation of peroxynitrite (ONOO−), a potent oxidant and nitrating agent . The production of ONOO− can lead to alterations in signaling pathways, impaired function, toxicity, and lipid peroxidation .
Pharmacokinetics
It is known that the extent of tyrosine nitration can be quantified by measuring 3-nitrotyrosine in biological matrices, such as blood, urine, and tissue .
Result of Action
The nitration of tyrosine residues by this compound can lead to alterations in protein function and signaling pathways . For example, nitration of Tyr385 of cyclooxygenase (COX) by using a very high molar excess of synthetic peroxynitrite over COX is associated with little loss of activity .
Action Environment
The action of this compound is influenced by various environmental factors. For instance, the presence of thiols can inhibit the formation of 3-nitro-L-tyrosine, while the presence of CO2 (usually supplied as bicarbonate) can elevate it
Analyse Biochimique
Biochemical Properties
3-Nitro-L-tyrosine-d3 interacts with a variety of enzymes, proteins, and other biomolecules. Reactive-nitrogen species, such as peroxynitrite (ONOO−) and nitryl chloride (NO2Cl), react with the aromatic ring of tyrosine in soluble amino acids and in proteins to form 3-nitrotyrosine . The extent of nitration can be quantified by measuring 3-nitrotyrosine in biological matrices, such as blood, urine, and tissue .
Cellular Effects
This compound has significant effects on various types of cells and cellular processes. It can affect protein behavior during neurodegenerative processes, such as those associated with Alzheimer’s and Parkinson’s diseases . It also influences cell function, including any impact on cell signaling pathways, gene expression, and cellular metabolism .
Molecular Mechanism
The molecular mechanism of this compound involves its interactions at the molecular level. It exerts its effects through binding interactions with biomolecules, enzyme inhibition or activation, and changes in gene expression .
Temporal Effects in Laboratory Settings
In laboratory settings, the effects of this compound change over time. This includes information on the product’s stability, degradation, and any long-term effects on cellular function observed in in vitro or in vivo studies .
Metabolic Pathways
This compound is involved in various metabolic pathways. It interacts with enzymes or cofactors and can have effects on metabolic flux or metabolite levels .
Méthodes De Préparation
Synthetic Routes and Reaction Conditions: The synthesis of (2S)-2-Amino-3-(2,3,6-trideuterio-4-hydroxy-5-nitrophenyl)propanoic acid typically involves multiple steps, starting from commercially available precursors. One common approach is to introduce the deuterium atoms through a deuterium exchange reaction. This can be achieved by treating the precursor with deuterated reagents under specific conditions. The hydroxyl and nitro groups are then introduced through nitration and hydroxylation reactions, respectively.
Industrial Production Methods: Industrial production of this compound may involve large-scale deuterium exchange reactions followed by nitration and hydroxylation. The process requires careful control of reaction conditions to ensure high yield and purity. The use of deuterated solvents and reagents is essential to achieve the desired isotopic labeling.
Analyse Des Réactions Chimiques
Types of Reactions:
Oxidation: The hydroxyl group can be oxidized to form a ketone or aldehyde.
Reduction: The nitro group can be reduced to an amino group using reducing agents like hydrogen gas in the presence of a catalyst.
Substitution: The aromatic ring can undergo electrophilic substitution reactions, such as halogenation or sulfonation.
Common Reagents and Conditions:
Oxidation: Potassium permanganate (KMnO4) or chromium trioxide (CrO3) in acidic conditions.
Reduction: Hydrogen gas (H2) with a palladium catalyst (Pd/C) or tin(II) chloride (SnCl2) in hydrochloric acid.
Substitution: Halogens (Cl2, Br2) in the presence of a Lewis acid catalyst like aluminum chloride (AlCl3).
Major Products:
Oxidation: Formation of ketones or aldehydes.
Reduction: Formation of aromatic amines.
Substitution: Formation of halogenated or sulfonated aromatic compounds.
Comparaison Avec Des Composés Similaires
Phenylalanine: The non-deuterated analog with similar structural features but different isotopic composition.
Tyrosine: Contains a hydroxyl group on the phenyl ring but lacks the nitro group.
Nitrophenylalanine: Similar to the compound but without deuterium atoms.
Uniqueness:
- The presence of deuterium atoms makes (2S)-2-Amino-3-(2,3,6-trideuterio-4-hydroxy-5-nitrophenyl)propanoic acid unique in terms of its isotopic labeling, which can significantly alter its physical and chemical properties.
- The combination of hydroxyl and nitro groups on the phenyl ring provides distinct reactivity and interaction profiles compared to other similar compounds.
This detailed article provides a comprehensive overview of (2S)-2-Amino-3-(2,3,6-trideuterio-4-hydroxy-5-nitrophenyl)propanoic acid, covering its preparation methods, chemical reactions, scientific research applications, mechanism of action, and comparison with similar compounds
Activité Biologique
3-Nitro-L-tyrosine-d3 is a deuterated derivative of 3-nitro-L-tyrosine, an important compound in biochemical research due to its role as a biomarker for oxidative stress and its involvement in various pathological conditions. This article explores the biological activity of this compound, focusing on its mechanisms of action, cellular effects, and implications in disease processes.
Chemical Structure and Properties
Chemical Formula: CHNO
Molecular Weight: 226.1861 g/mol
Structure: this compound contains a nitro group at the 3-position on the phenyl ring of L-tyrosine, which alters its biochemical interactions significantly compared to its non-nitrated counterpart.
The primary mechanism by which this compound exerts its biological activity involves the nitration of tyrosine residues in proteins. This process is catalyzed by reactive nitrogen species (RNS), particularly peroxynitrite (ONOO−). The nitration modifies the properties of tyrosine, impacting protein function and signaling pathways:
- Target Enzymes: One key target is glutathione reductase, a mitochondrial enzyme crucial for maintaining cellular redox balance. Nitration can inhibit this enzyme, leading to increased oxidative stress within cells .
- Biochemical Pathways: The formation of this compound is part of broader biochemical pathways involving nitric oxide (NO) and reactive oxygen species (ROS), which are often elevated during inflammatory responses .
Cellular Effects
The impact of this compound on cellular processes is profound:
- Neurodegenerative Diseases: Studies have shown that nitrated proteins are prevalent in neurodegenerative diseases such as Alzheimer's and Parkinson's disease. The presence of this compound can disrupt normal cellular signaling and promote neuroinflammation .
- Oxidative Stress Biomarker: It serves as a biomarker for oxidative stress, with elevated levels found in various diseases, including cardiovascular diseases, diabetes, and cancer. The detection of 3-nitro-L-tyrosine in biological samples can indicate underlying oxidative damage .
Case Studies and Research Findings
- Oxidative Stress in Disease:
- Protein Function Alteration:
- In Vivo Studies:
Summary Table: Biological Activity Highlights
Aspect | Details |
---|---|
Chemical Structure | CHNO |
Molecular Weight | 226.1861 g/mol |
Mechanism | Nitration by reactive nitrogen species |
Key Targets | Glutathione reductase |
Pathological Relevance | Biomarker for oxidative stress |
Associated Diseases | Alzheimer's, Parkinson's, cardiovascular |
Research Applications | Protein function studies, disease biomarkers |
Propriétés
IUPAC Name |
(2S)-2-amino-3-(2,3,6-trideuterio-4-hydroxy-5-nitrophenyl)propanoic acid | |
---|---|---|
Source | PubChem | |
URL | https://pubchem.ncbi.nlm.nih.gov | |
Description | Data deposited in or computed by PubChem | |
InChI |
InChI=1S/C9H10N2O5/c10-6(9(13)14)3-5-1-2-8(12)7(4-5)11(15)16/h1-2,4,6,12H,3,10H2,(H,13,14)/t6-/m0/s1/i1D,2D,4D | |
Source | PubChem | |
URL | https://pubchem.ncbi.nlm.nih.gov | |
Description | Data deposited in or computed by PubChem | |
InChI Key |
FBTSQILOGYXGMD-UOCCHMHCSA-N | |
Source | PubChem | |
URL | https://pubchem.ncbi.nlm.nih.gov | |
Description | Data deposited in or computed by PubChem | |
Canonical SMILES |
C1=CC(=C(C=C1CC(C(=O)O)N)[N+](=O)[O-])O | |
Source | PubChem | |
URL | https://pubchem.ncbi.nlm.nih.gov | |
Description | Data deposited in or computed by PubChem | |
Isomeric SMILES |
[2H]C1=C(C(=C(C(=C1C[C@@H](C(=O)O)N)[2H])[N+](=O)[O-])O)[2H] | |
Source | PubChem | |
URL | https://pubchem.ncbi.nlm.nih.gov | |
Description | Data deposited in or computed by PubChem | |
Molecular Formula |
C9H10N2O5 | |
Source | PubChem | |
URL | https://pubchem.ncbi.nlm.nih.gov | |
Description | Data deposited in or computed by PubChem | |
Molecular Weight |
229.20 g/mol | |
Source | PubChem | |
URL | https://pubchem.ncbi.nlm.nih.gov | |
Description | Data deposited in or computed by PubChem | |
Avertissement et informations sur les produits de recherche in vitro
Veuillez noter que tous les articles et informations sur les produits présentés sur BenchChem sont destinés uniquement à des fins informatives. Les produits disponibles à l'achat sur BenchChem sont spécifiquement conçus pour des études in vitro, qui sont réalisées en dehors des organismes vivants. Les études in vitro, dérivées du terme latin "in verre", impliquent des expériences réalisées dans des environnements de laboratoire contrôlés à l'aide de cellules ou de tissus. Il est important de noter que ces produits ne sont pas classés comme médicaments et n'ont pas reçu l'approbation de la FDA pour la prévention, le traitement ou la guérison de toute condition médicale, affection ou maladie. Nous devons souligner que toute forme d'introduction corporelle de ces produits chez les humains ou les animaux est strictement interdite par la loi. Il est essentiel de respecter ces directives pour assurer la conformité aux normes légales et éthiques en matière de recherche et d'expérimentation.