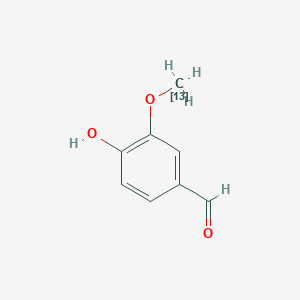
4-Hydroxy-3-(methoxy-13C)benzaldehyde
Vue d'ensemble
Description
4-Hydroxy-3-(methoxy-13C)benzaldehyde, also known as 3-Hydroxyanisaldehyde or Isovanillin, is an organic compound with the molecular formula HOC6H3(OCH3)CHO . It has a molecular weight of 152.15 .
Synthesis Analysis
Efficient synthetic procedures for the preparation of aroyl hydrazones were developed by converting the corresponding ethyl ester into hydrazides, followed by a reaction with 4-hydroxy-3-methoxy-benzaldehyde . A series of aromatic hydrazides and aroyl hydrazones were characterized by elemental analysis, IR, 1H NMR .Molecular Structure Analysis
The molecular structure of 4-Hydroxy-3-(methoxy-13C)benzaldehyde can be viewed using Java or Javascript .Chemical Reactions Analysis
4-Hydroxy-3-methoxy-benzaldehyde undergoes condensation with furan-2-carboxylic acid hydrazide and thiophene-2-carboxylic acid hydrazide to yield Schiff-bases . It also undergoes condensation reaction with 1-azabicyclo [2.2.2]octan-3-one to give (Z)-2- (3-hydroxy-4-methoxybenzylidene)-1-azabicyclo [2.2.2]octan-3-one .Physical And Chemical Properties Analysis
4-Hydroxy-3-(methoxy-13C)benzaldehyde has a boiling point of 179 °C/15 mmHg and a melting point of 113-115 °C .Applications De Recherche Scientifique
Food Authenticity and Quality Control
Vanillin-13C can be employed in isotopic analyses to authenticate the origin of vanillin in food products. By using 13C-NMR spectroscopy , researchers can determine the carbon stable isotope ratio and distinguish between natural and artificial vanillin . This is crucial for ensuring the quality and authenticity of food products, especially in industries where the source of vanillin is a marker of product quality.
Nonlinear Optical Materials
Vanillin derivatives, including those labeled with carbon-13, are explored for their potential in nonlinear optical applications . They are investigated for their third harmonic generation capabilities, which are important for developing new materials for lasers and optical data storage .
Antioxidant Properties
Vanillin-13C is studied for its antioxidant properties . It is reported to be a potent scavenger of reactive oxygen species (ROS), which is significant in research focused on oxidative stress and related diseases .
Authentication of Natural Products
Vanillin-13C plays a critical role in the authentication of natural products . By analyzing the isotopic distribution of carbon-13 in vanillin extracted from natural sources, researchers can confirm the natural origin of products and detect adulteration .
Safety and Hazards
Orientations Futures
Mécanisme D'action
Target of Action
Vanillin-13C, also known as 4-Hydroxy-3-(methoxy-13C)benzaldehyde, is a labeled form of Vanillin . Vanillin is a single molecule extracted from vanilla beans and is widely used in perfume, food, and medicine . The primary targets of Vanillin-13C are metabolic enzymes and proteases .
Mode of Action
Vanillin-13C exhibits a quorum-quenching mode of action . It interacts with its targets by docking towards similar amino acids (Thr 131, Ilu 214) responsible for autoinducer signal anchoring in the transcriptional regulatory proteins . This interaction disrupts the normal functioning of these proteins, leading to changes in the metabolic processes they regulate.
Biochemical Pathways
Vanillin-13C affects several biochemical pathways. It is involved in the vanillin biosynthetic pathway, where it can either be the product of the pathway or a precursor to other intermediates . The biosynthesis of vanillin involves crucial biochemical steps such as side-chain shortening, methylation, and glucoside formation .
Pharmacokinetics
The pharmacokinetics of Vanillin-13C involves its absorption, distribution, metabolism, and excretion (ADME). Stable heavy isotopes of hydrogen, carbon, and other elements have been incorporated into drug molecules, largely as tracers for quantitation during the drug development process . Deuteration has gained attention because of its potential to affect the pharmacokinetic and metabolic profiles of drugs .
Result of Action
The molecular and cellular effects of Vanillin-13C’s action are primarily related to its impact on metabolic enzymes and proteases . By disrupting the normal functioning of these proteins, Vanillin-13C can influence a variety of cellular processes, including metabolism and signal transduction.
Action Environment
The action, efficacy, and stability of Vanillin-13C can be influenced by various environmental factors. For instance, the isotopic distribution of 13C in Vanillin-13C can be used to determine its origin . This makes it attractive for the analysis in the same experiment of site-specific and total isotope effects for testing authenticity, quality, and typicality of food samples .
Propriétés
IUPAC Name |
4-hydroxy-3-(113C)methoxybenzaldehyde | |
---|---|---|
Source | PubChem | |
URL | https://pubchem.ncbi.nlm.nih.gov | |
Description | Data deposited in or computed by PubChem | |
InChI |
InChI=1S/C8H8O3/c1-11-8-4-6(5-9)2-3-7(8)10/h2-5,10H,1H3/i1+1 | |
Source | PubChem | |
URL | https://pubchem.ncbi.nlm.nih.gov | |
Description | Data deposited in or computed by PubChem | |
InChI Key |
MWOOGOJBHIARFG-OUBTZVSYSA-N | |
Source | PubChem | |
URL | https://pubchem.ncbi.nlm.nih.gov | |
Description | Data deposited in or computed by PubChem | |
Canonical SMILES |
COC1=C(C=CC(=C1)C=O)O | |
Source | PubChem | |
URL | https://pubchem.ncbi.nlm.nih.gov | |
Description | Data deposited in or computed by PubChem | |
Isomeric SMILES |
[13CH3]OC1=C(C=CC(=C1)C=O)O | |
Source | PubChem | |
URL | https://pubchem.ncbi.nlm.nih.gov | |
Description | Data deposited in or computed by PubChem | |
Molecular Formula |
C8H8O3 | |
Source | PubChem | |
URL | https://pubchem.ncbi.nlm.nih.gov | |
Description | Data deposited in or computed by PubChem | |
DSSTOX Substance ID |
DTXSID50481345 | |
Record name | 4-Hydroxy-3-(methoxy-13C)benzaldehyde | |
Source | EPA DSSTox | |
URL | https://comptox.epa.gov/dashboard/DTXSID50481345 | |
Description | DSSTox provides a high quality public chemistry resource for supporting improved predictive toxicology. | |
Molecular Weight |
153.14 g/mol | |
Source | PubChem | |
URL | https://pubchem.ncbi.nlm.nih.gov | |
Description | Data deposited in or computed by PubChem | |
Product Name |
4-Hydroxy-3-(methoxy-13C)benzaldehyde | |
CAS RN |
86884-84-6 | |
Record name | 4-Hydroxy-3-(methoxy-13C)benzaldehyde | |
Source | EPA DSSTox | |
URL | https://comptox.epa.gov/dashboard/DTXSID50481345 | |
Description | DSSTox provides a high quality public chemistry resource for supporting improved predictive toxicology. | |
Record name | 86884-84-6 | |
Source | European Chemicals Agency (ECHA) | |
URL | https://echa.europa.eu/information-on-chemicals | |
Description | The European Chemicals Agency (ECHA) is an agency of the European Union which is the driving force among regulatory authorities in implementing the EU's groundbreaking chemicals legislation for the benefit of human health and the environment as well as for innovation and competitiveness. | |
Explanation | Use of the information, documents and data from the ECHA website is subject to the terms and conditions of this Legal Notice, and subject to other binding limitations provided for under applicable law, the information, documents and data made available on the ECHA website may be reproduced, distributed and/or used, totally or in part, for non-commercial purposes provided that ECHA is acknowledged as the source: "Source: European Chemicals Agency, http://echa.europa.eu/". Such acknowledgement must be included in each copy of the material. ECHA permits and encourages organisations and individuals to create links to the ECHA website under the following cumulative conditions: Links can only be made to webpages that provide a link to the Legal Notice page. | |
Synthesis routes and methods I
Procedure details
Synthesis routes and methods II
Procedure details
Synthesis routes and methods III
Procedure details
Synthesis routes and methods IV
Procedure details
Retrosynthesis Analysis
AI-Powered Synthesis Planning: Our tool employs the Template_relevance Pistachio, Template_relevance Bkms_metabolic, Template_relevance Pistachio_ringbreaker, Template_relevance Reaxys, Template_relevance Reaxys_biocatalysis model, leveraging a vast database of chemical reactions to predict feasible synthetic routes.
One-Step Synthesis Focus: Specifically designed for one-step synthesis, it provides concise and direct routes for your target compounds, streamlining the synthesis process.
Accurate Predictions: Utilizing the extensive PISTACHIO, BKMS_METABOLIC, PISTACHIO_RINGBREAKER, REAXYS, REAXYS_BIOCATALYSIS database, our tool offers high-accuracy predictions, reflecting the latest in chemical research and data.
Strategy Settings
Precursor scoring | Relevance Heuristic |
---|---|
Min. plausibility | 0.01 |
Model | Template_relevance |
Template Set | Pistachio/Bkms_metabolic/Pistachio_ringbreaker/Reaxys/Reaxys_biocatalysis |
Top-N result to add to graph | 6 |
Feasible Synthetic Routes
Q & A
Q1: Why is Vanillin-13C-formyl used as a model compound in lignin research?
A1: Lignin, a complex polymer found in plant cell walls, is composed of various aromatic monomers. [] Vanillin is a similar aromatic compound structurally related to these monomers. By selectively enriching vanillin with 13C at specific positions, like the formyl group in Vanillin-13C-formyl, researchers can use Nuclear Magnetic Resonance (NMR) spectroscopy to obtain more detailed information about the structure and bonding of lignin model compounds. [] This information helps in understanding the properties and behavior of lignin itself.
Q2: What specific information about lignin can be obtained using Vanillin-13C-formyl and NMR?
A2: The research paper focuses on determining coupling constants (J(CH) and J(CC)) in lignin model compounds using NMR. [] These constants provide valuable insights into the electronic environment and interactions between atoms within the molecule. By analyzing the NMR spectra of Vanillin-13C-formyl, researchers can determine the absolute values of these coupling constants, which are essential for understanding the structural features and conformational preferences of lignin model compounds. [] This knowledge contributes to a deeper understanding of lignin's complex structure and its role in plant cell walls.
Avertissement et informations sur les produits de recherche in vitro
Veuillez noter que tous les articles et informations sur les produits présentés sur BenchChem sont destinés uniquement à des fins informatives. Les produits disponibles à l'achat sur BenchChem sont spécifiquement conçus pour des études in vitro, qui sont réalisées en dehors des organismes vivants. Les études in vitro, dérivées du terme latin "in verre", impliquent des expériences réalisées dans des environnements de laboratoire contrôlés à l'aide de cellules ou de tissus. Il est important de noter que ces produits ne sont pas classés comme médicaments et n'ont pas reçu l'approbation de la FDA pour la prévention, le traitement ou la guérison de toute condition médicale, affection ou maladie. Nous devons souligner que toute forme d'introduction corporelle de ces produits chez les humains ou les animaux est strictement interdite par la loi. Il est essentiel de respecter ces directives pour assurer la conformité aux normes légales et éthiques en matière de recherche et d'expérimentation.