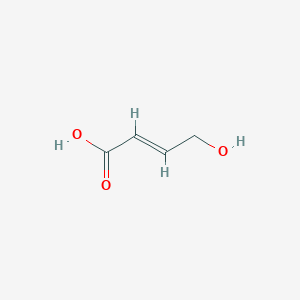
4-Hydroxycrotonic acid
Vue d'ensemble
Description
Synthesis Analysis
4-Hydroxycrotonic acid and its analogs have been synthesized through various methods, demonstrating the compound's accessibility for study and application. A notable method includes the convenient three-step synthesis from crotonic acid, which involves bromination, conversion to 4-aminocrotonic acid, and finally, obtaining 4-amino-3-hydroxybutyric acid under mild conditions (Pinza & Pifferi, 1978). Another synthesis approach involves the tritiation of trans 4-hydroxycrotonic acid (T-HCA) highlighting its structural significance and potential for biological study (Schmitt et al., 1989).
Molecular Structure Analysis
The crystal and molecular structure of 4-hydroxycrotonic acid and its analogs have been thoroughly analyzed, providing insight into their chemical behavior and interactions. For example, the crystal structures of trans-4-hydroxycrotonic acid (THCA) and its derivatives were determined using single-crystal X-ray diffraction techniques, revealing significant conformational details and the potential for low-energy states conducive to biological activity (Boulanger et al., 1987).
Chemical Reactions and Properties
4-Hydroxycrotonic acid undergoes various chemical reactions, including oxygenative and dehydrogenative [3 + 3] benzannulation reactions with α,β-unsaturated aldehydes and γ-phosphonyl crotonates, leading to the synthesis of 4-hydroxybiaryl-2-carboxylates. This process demonstrates the compound's versatility and potential for creating complex organic molecules (Joshi et al., 2016).
Physical Properties Analysis
The physical properties of 4-hydroxycrotonic acid, including its crystal structure and photodimerization behavior, have been studied to understand its stability and reactivity under various conditions. For instance, the photodimers obtained from 4-hydroxycrotonic acid γ-lactone under different conditions exhibit distinct anti-dimer forms, showcasing the compound's photochemical characteristics and the influence of physical state on its chemical behavior (Ohga & Matsuo, 1970).
Chemical Properties Analysis
The chemical properties of 4-hydroxycrotonic acid, including its involvement in lipid peroxidation and interactions with biomolecules, have been explored. Its reactivity and potential to form highly reactive aldehydes upon breakdown in biological systems indicate its importance in understanding oxidative stress and related cellular processes (Esterbauer et al., 1991).
Applications De Recherche Scientifique
Phenylpropanoid Metabolism : In a study by Mitra et al. (2002), 4-Hydroxycinnamoyl-CoA hydratase/lyase was expressed in Datura stramonium roots, leading to the formation of new compounds and potentially altering phenylpropanoid metabolism (Mitra et al., 2002).
Inhibition of Fatty Acid Oxidation : Olowe and Schulz (1982) found that 4-bromocrotonic acid effectively inhibits fatty acid oxidation and ketone body degradation in rat heart mitochondria, suggesting its role in beta-oxidation processes (Olowe & Schulz, 1982).
Functional and Structural Diversity in Bacteria : Burks et al. (2010) reported that a heterohexamer 4-oxalocrotonate tautomerase from Chloroflexus aurantiacus J-10-fl showed potential applications in bacterial utilization of aromatic hydrocarbons as carbon sources, highlighting functional and structural diversity in the tautomerase superfamily (Burks et al., 2010).
Biomanufacturing Applications : Li Fei-Fei et al. (2016) engineered an E. coli strain to efficiently produce 4-hydroxymandelic acid from lignocellulosic biomass, offering a promising route for biomanufacturing pharmaceuticals and food additives (Li Fei-Fei et al., 2016).
Intermediate for Value-Added Bioproducts : Wang et al. (2018) discussed the use of 4-Hydroxybenzoic acid as an intermediate for producing bioproducts like resveratrol, muconic acid, gastrodin, xiamenmycin, and vanillyl alcohol, applicable in food, cosmetics, pharmacy, and fungicides (Wang et al., 2018).
Reactivity and Biological Activities : Esterbauer et al. (1991) highlighted that compounds like 4-hydroxynonenal and related aldehydes formed by lipid peroxidation in biological systems exhibit various activities including cytotoxicity and chemotactic activity (Esterbauer et al., 1991).
Mécanisme D'action
Target of Action
The primary targets of (E)-4-Hydroxybut-2-enoic acid are yet to be definitively identified. The compound’s interaction with various biological targets is a subject of ongoing research. It’s important to note that the identification of a compound’s targets is a complex process that involves extensive biochemical and molecular biology studies .
Mode of Action
The mode of action of (E)-4-Hydroxybut-2-enoic acid is not fully understood at this time. The compound’s interaction with its targets and the resulting changes are subjects of ongoing research. Understanding the mode of action of a compound requires detailed studies on its binding to its targets, the conformational changes induced in the targets, and the downstream effects of these interactions .
Biochemical Pathways
The biochemical pathways affected by (E)-4-Hydroxybut-2-enoic acid are not fully known. The compound’s effects on various biochemical pathways depend on its targets and mode of action. Detailed studies on the compound’s effects on cellular metabolism, signal transduction, gene expression, and other biochemical processes are required to understand its role in these pathways .
Pharmacokinetics
The ADME (Absorption, Distribution, Metabolism, and Excretion) properties of (E)-4-Hydroxybut-2-enoic acid are not fully known. These properties determine the bioavailability of the compound, which is crucial for its biological activity. Studies on the compound’s absorption in the gastrointestinal tract, its distribution in the body, its metabolic transformations, and its excretion are required to understand its pharmacokinetics .
Result of Action
The molecular and cellular effects of (E)-4-Hydroxybut-2-enoic acid are not fully known. These effects depend on the compound’s targets, mode of action, and biochemical pathways affected. Detailed studies on the compound’s effects on cellular structures, functions, and viability are required to understand these effects .
Action Environment
Environmental factors can significantly influence the action, efficacy, and stability of (E)-4-Hydroxybut-2-enoic acid. Factors such as temperature, pH, presence of other compounds, and cellular environment can affect the compound’s stability, its interaction with its targets, and its overall biological activity .
Propriétés
IUPAC Name |
(E)-4-hydroxybut-2-enoic acid | |
---|---|---|
Source | PubChem | |
URL | https://pubchem.ncbi.nlm.nih.gov | |
Description | Data deposited in or computed by PubChem | |
InChI |
InChI=1S/C4H6O3/c5-3-1-2-4(6)7/h1-2,5H,3H2,(H,6,7)/b2-1+ | |
Source | PubChem | |
URL | https://pubchem.ncbi.nlm.nih.gov | |
Description | Data deposited in or computed by PubChem | |
InChI Key |
RMQJECWPWQIIPW-OWOJBTEDSA-N | |
Source | PubChem | |
URL | https://pubchem.ncbi.nlm.nih.gov | |
Description | Data deposited in or computed by PubChem | |
Canonical SMILES |
C(C=CC(=O)O)O | |
Source | PubChem | |
URL | https://pubchem.ncbi.nlm.nih.gov | |
Description | Data deposited in or computed by PubChem | |
Isomeric SMILES |
C(/C=C/C(=O)O)O | |
Source | PubChem | |
URL | https://pubchem.ncbi.nlm.nih.gov | |
Description | Data deposited in or computed by PubChem | |
Molecular Formula |
C4H6O3 | |
Source | PubChem | |
URL | https://pubchem.ncbi.nlm.nih.gov | |
Description | Data deposited in or computed by PubChem | |
DSSTOX Substance ID |
DTXSID901027169 | |
Record name | trans-4-hydroxycrotonic acid | |
Source | EPA DSSTox | |
URL | https://comptox.epa.gov/dashboard/DTXSID901027169 | |
Description | DSSTox provides a high quality public chemistry resource for supporting improved predictive toxicology. | |
Molecular Weight |
102.09 g/mol | |
Source | PubChem | |
URL | https://pubchem.ncbi.nlm.nih.gov | |
Description | Data deposited in or computed by PubChem | |
Physical Description |
Solid | |
Record name | 4-Hydroxycrotonic acid | |
Source | Human Metabolome Database (HMDB) | |
URL | http://www.hmdb.ca/metabolites/HMDB0003381 | |
Description | The Human Metabolome Database (HMDB) is a freely available electronic database containing detailed information about small molecule metabolites found in the human body. | |
Explanation | HMDB is offered to the public as a freely available resource. Use and re-distribution of the data, in whole or in part, for commercial purposes requires explicit permission of the authors and explicit acknowledgment of the source material (HMDB) and the original publication (see the HMDB citing page). We ask that users who download significant portions of the database cite the HMDB paper in any resulting publications. | |
Product Name |
4-Hydroxycrotonic acid | |
CAS RN |
24587-49-3, 4013-24-5 | |
Record name | trans-4-Hydroxycrotonic acid | |
Source | CAS Common Chemistry | |
URL | https://commonchemistry.cas.org/detail?cas_rn=24587-49-3 | |
Description | CAS Common Chemistry is an open community resource for accessing chemical information. Nearly 500,000 chemical substances from CAS REGISTRY cover areas of community interest, including common and frequently regulated chemicals, and those relevant to high school and undergraduate chemistry classes. This chemical information, curated by our expert scientists, is provided in alignment with our mission as a division of the American Chemical Society. | |
Explanation | The data from CAS Common Chemistry is provided under a CC-BY-NC 4.0 license, unless otherwise stated. | |
Record name | 4-Hydroxy-2-butenoic acid | |
Source | ChemIDplus | |
URL | https://pubchem.ncbi.nlm.nih.gov/substance/?source=chemidplus&sourceid=0004013245 | |
Description | ChemIDplus is a free, web search system that provides access to the structure and nomenclature authority files used for the identification of chemical substances cited in National Library of Medicine (NLM) databases, including the TOXNET system. | |
Record name | trans-4-hydroxycrotonic acid | |
Source | EPA DSSTox | |
URL | https://comptox.epa.gov/dashboard/DTXSID901027169 | |
Description | DSSTox provides a high quality public chemistry resource for supporting improved predictive toxicology. | |
Record name | 4-Hydroxycrotonic acid | |
Source | Human Metabolome Database (HMDB) | |
URL | http://www.hmdb.ca/metabolites/HMDB0003381 | |
Description | The Human Metabolome Database (HMDB) is a freely available electronic database containing detailed information about small molecule metabolites found in the human body. | |
Explanation | HMDB is offered to the public as a freely available resource. Use and re-distribution of the data, in whole or in part, for commercial purposes requires explicit permission of the authors and explicit acknowledgment of the source material (HMDB) and the original publication (see the HMDB citing page). We ask that users who download significant portions of the database cite the HMDB paper in any resulting publications. | |
Retrosynthesis Analysis
AI-Powered Synthesis Planning: Our tool employs the Template_relevance Pistachio, Template_relevance Bkms_metabolic, Template_relevance Pistachio_ringbreaker, Template_relevance Reaxys, Template_relevance Reaxys_biocatalysis model, leveraging a vast database of chemical reactions to predict feasible synthetic routes.
One-Step Synthesis Focus: Specifically designed for one-step synthesis, it provides concise and direct routes for your target compounds, streamlining the synthesis process.
Accurate Predictions: Utilizing the extensive PISTACHIO, BKMS_METABOLIC, PISTACHIO_RINGBREAKER, REAXYS, REAXYS_BIOCATALYSIS database, our tool offers high-accuracy predictions, reflecting the latest in chemical research and data.
Strategy Settings
Precursor scoring | Relevance Heuristic |
---|---|
Min. plausibility | 0.01 |
Model | Template_relevance |
Template Set | Pistachio/Bkms_metabolic/Pistachio_ringbreaker/Reaxys/Reaxys_biocatalysis |
Top-N result to add to graph | 6 |
Feasible Synthetic Routes
Q & A
Q1: What is the relationship between 4-hydroxycrotonic acid (T-HCA) and γ-hydroxybutyric acid (GHB)?
A1: 4-Hydroxycrotonic acid (T-HCA) is a naturally occurring substance in the central nervous system and is structurally similar to γ-hydroxybutyric acid (GHB). Research suggests that T-HCA may act as an endogenous ligand for the specific high-affinity binding sites that GHB interacts with. [, ] This suggests a potential competitive relationship between these two compounds. [, ]
Q2: What is the significance of developing high-affinity ligands for GHB binding sites?
A3: Developing high-affinity ligands like those derived from T-HCA holds significant potential for both target characterization and ligand identification for the high-affinity GHB binding sites. [] This could facilitate the development of new therapeutic agents targeting these sites.
Q3: Can you explain the metabolic pathway involving 1,4-butanediol, GHB, and T-HCA?
A4: 1,4-Butanediol (1,4-BD) is metabolized to GHB via alcohol dehydrogenase (ADH) and aldehyde dehydrogenase. [, ] GHB can be further metabolized to both γ-aminobutyric acid (GABA) and T-HCA. [, ] Both GHB and T-HCA exhibit pharmacological activity at GHB receptors, while GABA acts on GABAB receptors. [, ] This interconnected metabolic pathway highlights the complex pharmacological effects associated with these compounds.
Q4: Has T-HCA been successfully labeled for use in research?
A6: Yes, T-HCA has been successfully tritiated at the 2,3 positions, resulting in T-HCA-[2,3-3H] with high specific radioactivity and radiochemical purity. [] This development enables researchers to further investigate the distribution, metabolism, and binding characteristics of T-HCA in biological systems.
Q5: Beyond its relationship with GHB, are there other applications for 4-hydroxycrotonic acid?
A7: 4-Hydroxycrotonic acid serves as a key component in synthesizing a phosphorus-free crude oil metal chelating agent. [] This agent, comprising a terpolymer incorporating 4-hydroxycrotonic acid, exhibits excellent metal chelating performance and is considered environmentally friendly. [] This application highlights the versatility of 4-hydroxycrotonic acid in various chemical processes.
Q6: What are the implications of the structural analysis of T-HCA and its analogs?
A8: Crystal and molecular structure analysis of T-HCA and its analogs, such as trans-4-hydroxy-4-o-chlorophenylcrotonic acid (THCCA) and trans-4-hydroxy-4-p-nitrophenylcrotonic acid (THNCA), provides valuable insights into the spatial arrangement of atoms within the molecule. [] This information is crucial for understanding the structure-activity relationship and designing new analogs with improved pharmacological properties.
Avertissement et informations sur les produits de recherche in vitro
Veuillez noter que tous les articles et informations sur les produits présentés sur BenchChem sont destinés uniquement à des fins informatives. Les produits disponibles à l'achat sur BenchChem sont spécifiquement conçus pour des études in vitro, qui sont réalisées en dehors des organismes vivants. Les études in vitro, dérivées du terme latin "in verre", impliquent des expériences réalisées dans des environnements de laboratoire contrôlés à l'aide de cellules ou de tissus. Il est important de noter que ces produits ne sont pas classés comme médicaments et n'ont pas reçu l'approbation de la FDA pour la prévention, le traitement ou la guérison de toute condition médicale, affection ou maladie. Nous devons souligner que toute forme d'introduction corporelle de ces produits chez les humains ou les animaux est strictement interdite par la loi. Il est essentiel de respecter ces directives pour assurer la conformité aux normes légales et éthiques en matière de recherche et d'expérimentation.