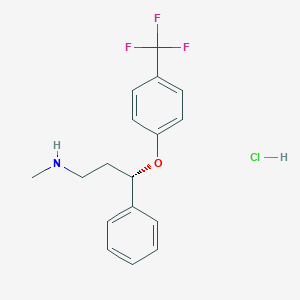
(S)-Fluoxetine Hydrochloride
Vue d'ensemble
Description
(S)-Fluoxetine Hydrochloride is the pharmacologically active enantiomer of fluoxetine hydrochloride, a selective serotonin reuptake inhibitor (SSRI) first developed by Eli Lilly in the 1970s and approved by the FDA in 1987 for major depressive disorder . Unlike the racemic mixture (R- and S-enantiomers), the (S)-enantiomer exhibits enhanced binding affinity to the serotonin transporter (SERT), contributing to its efficacy in treating depression, obsessive-compulsive disorder (OCD), panic disorder, and bulimia nervosa . Structurally, it is a bicyclic compound with a trifluoromethylphenyl group, distinguishing it from traditional tricyclic antidepressants (TCAs) and monoamine oxidase inhibitors (MAOIs) .
Pharmacokinetically, this compound has a prolonged half-life (4–6 days) due to its active metabolite, norfluoxetine, which extends therapeutic effects and allows once-daily dosing . Its safety profile is superior to TCAs, with reduced anticholinergic and cardiovascular side effects, making it a first-line treatment for depression .
Mécanisme D'action
Target of Action
S-(+)-Fluoxetine hydrochloride, also known as (S)-Fluoxetine Hydrochloride, primarily targets the serotonin transporter (SERT) . SERT is a type of monoamine transporter protein that transports serotonin from the synaptic cleft to the presynaptic neuron . This reuptake of serotonin by presynaptic neurons allows the recycling of this neurotransmitter and terminates its action, thus regulating mood .
Mode of Action
S-(+)-Fluoxetine hydrochloride acts as a selective serotonin reuptake inhibitor (SSRI) . This results in serotonin staying in the synaptic cleft for a longer period of time than usual, which can lead to prolonged stimulation of the postsynaptic neuron .
Biochemical Pathways
The increased serotonin in the synaptic cleft enhances serotonin stimulation of the postsynaptic neuron. This leads to downstream effects in several biochemical pathways, particularly those involved in mood regulation . These pathways include the serotonergic pathway , which is directly affected by increased serotonin levels, and the dopaminergic and noradrenergic pathways , which are indirectly influenced through serotonin’s modulatory effects .
Pharmacokinetics
The ADME (Absorption, Distribution, Metabolism, and Excretion) properties of S-(+)-Fluoxetine hydrochloride are crucial for its bioavailability . After oral administration, it is well absorbed from the gastrointestinal tract, widely distributed throughout the body, and extensively metabolized in the liver . It is primarily excreted in the urine . The half-life of S-(+)-Fluoxetine hydrochloride is relatively long, allowing for once-daily dosing .
Result of Action
The molecular and cellular effects of S-(+)-Fluoxetine hydrochloride’s action primarily involve changes in neuronal signaling and function due to increased serotonin levels . This can lead to enhanced neural plasticity and changes in the activity of neural circuits involved in mood regulation . At a cellular level, it can affect various processes such as cell growth, survival, and synaptic plasticity .
Action Environment
The action, efficacy, and stability of S-(+)-Fluoxetine hydrochloride can be influenced by various environmental factors. For instance, the drug’s absorption can be affected by the presence of food in the stomach . Its metabolism can be influenced by factors such as the individual’s age, liver function, and genetic makeup . Furthermore, research suggests that the living environment can influence the antidepressant effects of SSRIs, including fluoxetine .
Analyse Biochimique
Biochemical Properties
S-(+)-Fluoxetine hydrochloride acts primarily by inhibiting the reuptake of serotonin in the synaptic cleft, thereby increasing the concentration of serotonin available for binding to the post-synaptic receptor . This compound interacts with the serotonin transporter (SERT), a protein responsible for the reuptake of serotonin from the synaptic cleft . The interaction between S-(+)-Fluoxetine hydrochloride and SERT is characterized by the binding of the compound to the transporter, inhibiting its function and leading to an increase in serotonin levels .
Cellular Effects
S-(+)-Fluoxetine hydrochloride has a significant impact on various types of cells and cellular processes. It influences cell function by modulating the serotonergic system, which plays a crucial role in numerous cellular processes, including cell signaling pathways, gene expression, and cellular metabolism . For instance, the increased serotonin levels resulting from the action of S-(+)-Fluoxetine hydrochloride can influence the activity of various serotonin receptors, which are involved in numerous cellular signaling pathways .
Molecular Mechanism
The molecular mechanism of action of S-(+)-Fluoxetine hydrochloride involves its binding to the serotonin transporter (SERT), inhibiting the reuptake of serotonin . This leads to an increase in the concentration of serotonin in the synaptic cleft, enhancing serotonin signaling. The compound does not directly activate or inhibit enzymes but influences enzyme activity indirectly through its effects on serotonin levels .
Temporal Effects in Laboratory Settings
The effects of S-(+)-Fluoxetine hydrochloride change over time in laboratory settings. The compound is stable under standard conditions, and its effects on cellular function are typically observed following acute and chronic administration . Long-term effects on cellular function have been observed in in vitro and in vivo studies, with chronic administration leading to adaptive changes in the serotonergic system .
Dosage Effects in Animal Models
The effects of S-(+)-Fluoxetine hydrochloride vary with different dosages in animal models. Lower doses typically result in a significant increase in serotonin levels, while higher doses can lead to a saturation of the serotonin transporter, beyond which no further increase in serotonin levels is observed . High doses of S-(+)-Fluoxetine hydrochloride can also lead to side effects, including changes in behavior and physiology .
Metabolic Pathways
S-(+)-Fluoxetine hydrochloride is metabolized primarily in the liver, mainly through the CYP2D6 enzyme . The primary metabolite is norfluoxetine, which also exhibits SSRI activity . This metabolic pathway involves the conversion of S-(+)-Fluoxetine hydrochloride to norfluoxetine through N-demethylation .
Transport and Distribution
S-(+)-Fluoxetine hydrochloride is well-absorbed following oral administration and is distributed throughout the body . It is highly bound to plasma proteins, which can influence its distribution . The compound can cross the blood-brain barrier, allowing it to exert its effects on the central nervous system .
Subcellular Localization
S-(+)-Fluoxetine hydrochloride, being a lipophilic compound, can diffuse across cell membranes and reach various subcellular compartments. Its primary site of action is the presynaptic neuron, where it binds to the serotonin transporter located on the presynaptic membrane . By inhibiting the function of this transporter, S-(+)-Fluoxetine hydrochloride increases the concentration of serotonin in the synaptic cleft, enhancing serotonin signaling .
Activité Biologique
(S)-Fluoxetine Hydrochloride, commonly known as fluoxetine, is a selective serotonin reuptake inhibitor (SSRI) widely used in the treatment of various mood disorders, including major depressive disorder, obsessive-compulsive disorder (OCD), and bulimia nervosa. This article delves into the biological activity of (S)-fluoxetine, focusing on its pharmacological mechanisms, metabolic pathways, and clinical implications based on diverse research findings.
Pharmacological Mechanisms
(S)-Fluoxetine primarily functions by inhibiting the reuptake of serotonin (5-HT) in the synaptic cleft, thereby increasing its availability in the brain. This mechanism is crucial for its antidepressant effects. The selectivity for serotonin over other neurotransmitters distinguishes fluoxetine from tricyclic antidepressants (TCAs), which affect multiple neurotransmitter systems.
Key Findings:
- Inhibition of 5-HT Uptake : Fluoxetine has been shown to significantly inhibit the [3H]-5-HT uptake process in both animal and human models. Studies indicate that fluoxetine reduces 5-HT uptake by approximately 56% in nerve endings from treated rats, demonstrating its potency as an SSRI .
- Enantiomeric Activity : Fluoxetine exists as a racemic mixture of (S)- and (R)-enantiomers. The (S)-enantiomer is predominantly responsible for its pharmacological effects due to its slower elimination rate and higher plasma concentration at steady state compared to the (R)-enantiomer .
Metabolism and Pharmacokinetics
Fluoxetine undergoes extensive hepatic metabolism primarily via cytochrome P450 enzymes, particularly CYP2D6, CYP2C19, and CYP3A4. The active metabolite, norfluoxetine, also exhibits significant biological activity.
Metabolic Pathways:
- Demethylation to Norfluoxetine : The conversion of fluoxetine to norfluoxetine is stereoselective towards the (S)-enantiomer, which has a longer half-life (7-10 days) compared to fluoxetine's half-life of 1-4 days .
- CYP2D6 Inhibition : Both fluoxetine and norfluoxetine are potent inhibitors of CYP2D6, which can lead to drug interactions with other medications metabolized by this enzyme .
Clinical Efficacy and Safety
Numerous clinical studies have confirmed the efficacy of fluoxetine in treating depression and anxiety disorders. A meta-analysis involving over 9,000 patients demonstrated that fluoxetine is effective from the first week of therapy with a favorable safety profile compared to TCAs .
Efficacy Data:
- Depression Treatment : Fluoxetine has shown significant improvement in depressive symptoms with fewer patients discontinuing treatment due to adverse effects compared to TCAs .
- Safety Profile : Common side effects include nausea, insomnia, and diarrhea; however, serious adverse events are rare. Notably, fluoxetine does not significantly increase suicide risk among treated populations .
Case Studies
Several case studies illustrate both the therapeutic benefits and potential misuse of fluoxetine:
- Fluoxetine Abuse Case : A report highlighted a patient who misused fluoxetine during a family crisis, experiencing increased energy and mood elevation but also numbness and calmness. This case underscores the potential for misuse in vulnerable populations .
- Teenage Misuse : Another case involved a teenager who resorted to snorting fluoxetine capsules as a coping mechanism for stress. Despite temporary mood improvements, this behavior raises concerns about the drug's misuse potential among adolescents .
Research Findings Summary
Applications De Recherche Scientifique
Therapeutic Indications
Fluoxetine has received FDA approval for several conditions:
- Major Depressive Disorder (MDD) : Approved for individuals aged 8 and older.
- Obsessive-Compulsive Disorder (OCD) : Approved for individuals aged 7 and older.
- Panic Disorder : Can be prescribed with or without agoraphobia.
- Bulimia Nervosa : Recognized as effective in managing this eating disorder.
- Bipolar Disorder : Used as an adjunct treatment for depressive episodes.
- Treatment-Resistant Depression : Often combined with olanzapine for enhanced efficacy.
In addition to these approved uses, fluoxetine is employed off-label for conditions such as binge eating disorder, social anxiety disorder, premenstrual dysphoric disorder, borderline personality disorder, and post-traumatic stress disorder (PTSD) .
Emerging Research Findings
Recent studies have expanded our understanding of fluoxetine's effects beyond mood disorders. Notable findings include:
- Cognitive Function Enhancement : Research indicates that fluoxetine can improve cognitive functions by modulating synaptic plasticity. It has been shown to increase dendritic spine density in the hippocampus and medial prefrontal cortex, which are critical areas for learning and memory .
- Neurogenesis Promotion : Fluoxetine stimulates neurogenesis in the hippocampus, counteracting declines associated with chronic stress. This effect is linked to increased levels of Brain-Derived Neurotrophic Factor (BDNF), which supports neuronal growth and survival .
- Neuroprotective Effects : In animal models of neurodegenerative diseases such as Alzheimer's, fluoxetine treatment has resulted in enhanced synapse formation and stability, suggesting potential benefits in neuroprotection .
- Age and Region-Specific Effects : The impact of fluoxetine on dendritic structure varies with age and brain region. For instance, it reduces dendritic complexity in adult cortical regions while enhancing it in younger animals' dentate gyrus .
Case Studies
Several case studies illustrate the diverse applications of fluoxetine:
- Case Study 1 : A clinical trial involving patients with MDD demonstrated that fluoxetine significantly reduced depressive symptoms compared to placebo after 12 weeks of treatment.
- Case Study 2 : In a study focusing on patients with bulimia nervosa, fluoxetine was shown to reduce binge-eating episodes and purging behaviors effectively.
- Case Study 3 : Research on PTSD patients indicated that fluoxetine led to significant improvements in symptom severity when combined with cognitive behavioral therapy.
Analyse Des Réactions Chimiques
Key Synthetic Routes
(S)-Fluoxetine hydrochloride is synthesized through stereoselective methods to achieve its specific configuration. Below are two prominent routes:
Asymmetric Carbonyl-Ene Reaction
Developed by Miles et al. , this method achieves 99% enantiomeric excess (ee):
- Reaction : Benzaldehyde undergoes an asymmetric ene reaction with 3-methylene-2,3-dihydrofuran using a Ti(OiPr)₄/(R)-BINOL catalyst.
- Epoxide Formation : Intermediate diol is converted to an epoxide.
- Ring Opening : Epoxide reacts with methylamine to yield (S)-3-methylamino-1-phenylpropanol.
- Phenoxy Substitution : Nucleophilic aromatic substitution with 4-trifluoromethylphenol under basic conditions (DMSO, 80–100°C).
- Hydrochloride Formation : Acidification with HCl yields the final product .
Key Metrics :
Nucleophilic Aromatic Substitution
- Starting Material : N-Methyl-3-hydroxy-3-phenylpropylamine.
- Coupling : Reaction with 4-iodobenzotrifluoride in xylenes (130°C, CuBr/Cs₂CO₃).
- Workup : Purification with methyl t-butyl ether and HCl/isopropanol precipitation.
- Yield : 2.4 g (72% based on 10 mmol scale) .
Metabolic Reactions
(S)-Fluoxetine undergoes extensive hepatic metabolism mediated by cytochrome P450 enzymes :
Phase I Metabolism
- Demethylation : CYP2D6, CYP3A4, and CYP2C9 convert (S)-fluoxetine to (S)-norfluoxetine (active metabolite) .
- O-Dealkylation : CYP2C19 and CYP3A4 produce para-trifluoromethylphenol, further metabolized to hippuric acid .
Phase II Metabolism
- Glucuronidation : Both (S)-fluoxetine and (S)-norfluoxetine are conjugated via UGT1A9/2B7 for renal excretion .
Stability and Degradation
- Hydrolysis : Cleavage of the ether bond, generating 4-trifluoromethylphenol and 3-methylamino-1-phenylpropanol .
- Oxidation : Formation of N-oxide derivatives under strongly acidic conditions .
Drug-Drug Interaction Mechanisms
Q & A
Basic Research Questions
Q. How can researchers determine the solubility of (S)-Fluoxetine Hydrochloride in supercritical CO₂ for drug formulation studies?
- Methodological Answer : Quantitative Structure-Property Relationship (QSPR) models, such as Support Vector Regression (SVR), can predict solubility using molecular descriptors (e.g., density, temperature, and pressure). Experimental validation involves measuring solubility via high-pressure equilibrium cells and comparing results with computational predictions .
Q. What analytical methods are recommended for ensuring enantiomeric purity during chiral synthesis of this compound?
- Methodological Answer : Chiral High-Performance Liquid Chromatography (HPLC) or Supercritical Fluid Chromatography (SFC) with polysaccharide-based columns (e.g., Chiralpak®) can resolve enantiomers. Validation includes comparing retention times with pure (S)- and (R)-enantiomer standards and assessing peak symmetry .
Q. How should researchers convert between the free base and hydrochloride salt forms of fluoxetine in experimental formulations?
- Methodological Answer : Use a molar conversion factor of 1.12 (fluoxetine hydrochloride to fluoxetine free base). For example, to prepare 25 mg of fluoxetine free base, weigh 28 mg of fluoxetine hydrochloride. Validate purity via elemental analysis or NMR .
Q. What quality control protocols are critical for quantifying this compound in tablet formulations?
- Methodological Answer : Employ UV spectrophotometry (λ = 227 nm) or HPLC with a C18 column (mobile phase: acetonitrile-phosphate buffer, pH 3.0). Validate methods using USP reference standards and assess linearity (1–50 µg/mL), precision (RSD < 2%), and recovery (98–102%) .
Q. How can researchers assess the impact of co-crystallization on the physicochemical properties of this compound?
- Methodological Answer : Screen co-crystal formers (e.g., benzoic acid) via solvent evaporation or grinding. Characterize using Powder X-Ray Diffraction (PXRD) and Differential Scanning Calorimetry (DSC). Compare dissolution rates in simulated gastric fluid (e.g., intrinsic dissolution rate apparatus) .
Advanced Research Questions
Q. What strategies improve the dissolution rate and bioavailability of this compound through crystal engineering?
- Methodological Answer : Design cocrystals with organic acids (e.g., fumaric acid) to alter crystal lattice energy. Use cryo-EM or single-crystal X-ray diffraction to analyze structural changes. Conduct in vitro dissolution studies and correlate with in vivo pharmacokinetic data .
Q. How can in silico models predict the solubility and stability of this compound derivatives?
- Methodological Answer : Develop Artificial Neural Network (ANN) models using descriptors like LogP, molecular weight, and hydrogen-bonding capacity. Validate predictions with experimental solubility data in diverse solvents (e.g., ethanol, water) .
Q. What mechanisms underlie this compound's interaction with non-neurological targets, such as viral proteins?
- Methodological Answer : Use cryo-EM and ATPase activity assays to study binding to viral 2C AAA+ ATPases (e.g., coxsackievirus B3). Dose-response experiments (IC₅₀ determination) and molecular docking can identify allosteric binding sites .
Q. How can researchers resolve contradictions in pharmacokinetic data for this compound across preclinical models?
- Methodological Answer : Perform interspecies scaling using physiologically based pharmacokinetic (PBPK) modeling. Validate with in vivo studies in rodents and primates, measuring plasma half-life, clearance, and metabolite profiles (e.g., norfluoxetine) .
Q. What advanced techniques validate the chiral resolution of this compound in complex matrices (e.g., plasma)?
Comparaison Avec Des Composés Similaires
Structural and Mechanistic Differences
(S)-Fluoxetine Hydrochloride belongs to the SSRI class, which includes sertraline hydrochloride, paroxetine hydrochloride, and citalopram. Unlike serotonin-norepinephrine reuptake inhibitors (SNRIs, e.g., venlafaxine hydrochloride) or TCAs (e.g., amitriptyline hydrochloride), SSRIs selectively inhibit SERT with minimal impact on norepinephrine or dopamine transporters .
Compound | Class | Primary Target | Key Structural Feature |
---|---|---|---|
This compound | SSRI | Serotonin transporter | Trifluoromethylphenyl group, bicyclic backbone |
Sertraline Hydrochloride | SSRI | Serotonin transporter | Naphthylamine backbone |
Venlafaxine Hydrochloride | SNRI | SERT, NET | Phenoxyethylamine backbone |
Amitriptyline Hydrochloride | TCA | SERT, NET, mACh receptors | Tricyclic dibenzocycloheptene ring |
Ansofaxine Hydrochloride | TRI (novel) | Tyrosine kinase receptors | Not disclosed (under investigation) |
Pharmacological Efficacy
Antidepressant and Anti-Tumor Effects
In colon cancer cell lines (HCT116, SW620), this compound demonstrated dose-dependent proliferation inhibition comparable to novel triple reuptake inhibitors (TRIs) like ansofaxine hydrochloride and amitifadine hydrochloride. However, in mouse models (CT26, MCA38), all three compounds showed similar efficacy, suggesting interspecies variability in drug response .
Compound | IC50 (μM) in HCT116 | IC50 (μM) in SW620 |
---|---|---|
This compound | 12.3 ± 1.2 | 15.8 ± 1.5 |
Ansofaxine Hydrochloride | 10.9 ± 0.9 | 14.2 ± 1.1 |
Amitifadine Hydrochloride | 11.5 ± 1.0 | 13.7 ± 1.3 |
Gastroprotective Effects
This compound significantly reduced gastric mucosal injury in rat models of chronic psychological stress ulcers (CPSU) and chronic social defeat (CSD), outperforming non-SSRI antidepressants .
Side-Effect Profiles
A meta-analysis comparing SSRIs, SNRIs, and TCAs found this compound to have lower rates of sedation, weight gain, and anticholinergic effects than TCAs but higher incidence of insomnia and sexual dysfunction compared to sertraline .
Adverse Effect | (S)-Fluoxetine | Sertraline | Amitriptyline | Venlafaxine |
---|---|---|---|---|
Nausea | 21% | 25% | 15% | 35% |
Insomnia | 18% | 12% | 10% | 20% |
Sexual Dysfunction | 30% | 22% | 25% | 40% |
Dry Mouth | 10% | 8% | 45% | 15% |
Incidence rates from clinical trials .
Pharmacokinetics and Drug Interactions
Parameter | (S)-Fluoxetine | Paroxetine | Venlafaxine |
---|---|---|---|
Half-life (hours) | 96–144 | 21–24 | 5–11 |
CYP2D6 Inhibition | Strong | Moderate | None |
Protein Binding | 95% | 93% | 27% |
Data from pharmacokinetic studies .
Clinical and Commercial Impact
This compound remains a dominant antidepressant, with global sales exceeding $1.2 billion annually. Generic versions from Wockhardt and Dr. Reddy’s Laboratories account for 60% of the market, reflecting its cost-effectiveness compared to newer agents like vortioxetine .
Méthodes De Préparation
Key Synthetic Routes for (S)-Fluoxetine Hydrochloride
Alkylation of Chiral Amino Alcohols
The most widely utilized method involves the alkylation of (S)-N-methyl-3-hydroxy-3-(phenyl)propylamine with 1-chloro-4-(trifluoromethyl)benzene. This reaction is conducted in dimethylsulfoxide (DMSO) using alkaline metal hydroxides (e.g., KOH or NaOH) as bases .
Reaction Conditions:
-
Solvent: DMSO (polar aprotic solvent enhances nucleophilicity)
-
Base: KOH or NaOH (1.3–3.0 equivalents)
-
Temperature: 80–110°C
-
Time: 4–20 hours
Mechanism:
The hydroxide deprotonates the hydroxyl group of the amino alcohol, generating an alkoxide ion. This nucleophile undergoes an SN2 attack on the electron-deficient aromatic ring of 1-chloro-4-(trifluoromethyl)benzene, forming the ether linkage while retaining the S-configuration .
Example Protocol (Patent WO1994000416A1):
-
Dissolve 50 kg (±)-N-methyl-3-hydroxy-3-(phenyl)propylamine in 180 L DMSO.
-
Add 56.35 kg KOH (10.4% water) and stir at 100°C for 1 hour.
-
Introduce 56 L 1-chloro-4-(trifluoromethyl)benzene and react for 10 hours.
-
Quench with aqueous NaCl, extract with toluene, and isolate the hydrochloride salt via acidification .
Alternative Methods and Historical Context
Earlier syntheses relied on toxic reagents like cyanogen bromide for N-demethylation, which posed safety and scalability challenges . Modern approaches prioritize safer bases and solvents:
Stereochemical Control and Enantiomeric Purity
Preserving the S-configuration requires optically pure starting materials. (S)-N-methyl-3-hydroxy-3-(phenyl)propylamine is synthesized via asymmetric reduction of β-keto amines or resolution techniques . Key strategies include:
-
Chiral Pool Synthesis: Using enantiomerically enriched precursors derived from natural sources.
-
Catalytic Asymmetric Hydrogenation: Employing transition-metal catalysts (e.g., Ru-BINAP) to reduce imine intermediates .
Critical Factor:
Racemization is minimized by avoiding protic solvents and high temperatures (>120°C) during the alkylation step .
Optimization of Reaction Parameters
Base Selection
Potassium hydroxide outperforms sodium hydroxide due to its higher solubility in DMSO, which accelerates alkoxide formation. A 30% molar excess of KOH ensures complete deprotonation of the amino alcohol .
Solvent Effects
DMSO’s high polarity stabilizes the transition state, while its aprotic nature prevents side reactions. Substituting DMSO with N-methylpyrrolidone (NMP) reduces yields by 15–20% due to increased viscosity .
Temperature and Time
-
80–100°C: Optimal for balancing reaction rate and product stability.
-
>110°C: Risk of byproduct formation (e.g., elimination products).
-
<4 hours: Incomplete conversion; >20 hours: No significant yield improvement .
Industrial-Scale Production Considerations
The KOH/DMSO method is preferred for manufacturing due to:
-
Cost Efficiency: KOH ($0.50/kg) vs. NaH ($200/kg).
-
Safety: Eliminates explosion risks associated with sodium hydride.
-
Scalability: Batch sizes up to 500 kg have been reported with consistent yields .
Case Study:
A pilot plant using 50 kg batches achieved 87% yield with 99.5% enantiomeric excess (ee), confirmed by chiral HPLC .
Quality Control and Analytical Methods
Key Metrics:
-
Enantiomeric Purity: Chiral HPLC (Chiralpak AD-H column, hexane:isopropanol 90:10).
-
Chemical Purity: GC-MS (>99.5% by area normalization).
Regulatory Compliance:
-
ICH Q3A guidelines for impurities (<0.1% R-enantiomer).
-
USP <621> for residual solvents (DMSO <500 ppm).
Propriétés
IUPAC Name |
(3S)-N-methyl-3-phenyl-3-[4-(trifluoromethyl)phenoxy]propan-1-amine;hydrochloride | |
---|---|---|
Source | PubChem | |
URL | https://pubchem.ncbi.nlm.nih.gov | |
Description | Data deposited in or computed by PubChem | |
InChI |
InChI=1S/C17H18F3NO.ClH/c1-21-12-11-16(13-5-3-2-4-6-13)22-15-9-7-14(8-10-15)17(18,19)20;/h2-10,16,21H,11-12H2,1H3;1H/t16-;/m0./s1 | |
Source | PubChem | |
URL | https://pubchem.ncbi.nlm.nih.gov | |
Description | Data deposited in or computed by PubChem | |
InChI Key |
GIYXAJPCNFJEHY-NTISSMGPSA-N | |
Source | PubChem | |
URL | https://pubchem.ncbi.nlm.nih.gov | |
Description | Data deposited in or computed by PubChem | |
Canonical SMILES |
CNCCC(C1=CC=CC=C1)OC2=CC=C(C=C2)C(F)(F)F.Cl | |
Source | PubChem | |
URL | https://pubchem.ncbi.nlm.nih.gov | |
Description | Data deposited in or computed by PubChem | |
Isomeric SMILES |
CNCC[C@@H](C1=CC=CC=C1)OC2=CC=C(C=C2)C(F)(F)F.Cl | |
Source | PubChem | |
URL | https://pubchem.ncbi.nlm.nih.gov | |
Description | Data deposited in or computed by PubChem | |
Molecular Formula |
C17H19ClF3NO | |
Source | PubChem | |
URL | https://pubchem.ncbi.nlm.nih.gov | |
Description | Data deposited in or computed by PubChem | |
DSSTOX Substance ID |
DTXSID00872289 | |
Record name | (+)-(S)-Fluoxetine hydrochloride | |
Source | EPA DSSTox | |
URL | https://comptox.epa.gov/dashboard/DTXSID00872289 | |
Description | DSSTox provides a high quality public chemistry resource for supporting improved predictive toxicology. | |
Molecular Weight |
345.8 g/mol | |
Source | PubChem | |
URL | https://pubchem.ncbi.nlm.nih.gov | |
Description | Data deposited in or computed by PubChem | |
CAS No. |
114247-06-2 | |
Record name | (S)-Fluoxetine hydrochloride | |
Source | CAS Common Chemistry | |
URL | https://commonchemistry.cas.org/detail?cas_rn=114247-06-2 | |
Description | CAS Common Chemistry is an open community resource for accessing chemical information. Nearly 500,000 chemical substances from CAS REGISTRY cover areas of community interest, including common and frequently regulated chemicals, and those relevant to high school and undergraduate chemistry classes. This chemical information, curated by our expert scientists, is provided in alignment with our mission as a division of the American Chemical Society. | |
Explanation | The data from CAS Common Chemistry is provided under a CC-BY-NC 4.0 license, unless otherwise stated. | |
Record name | Fluoxetine hydrochloride, (S)- | |
Source | ChemIDplus | |
URL | https://pubchem.ncbi.nlm.nih.gov/substance/?source=chemidplus&sourceid=0114247062 | |
Description | ChemIDplus is a free, web search system that provides access to the structure and nomenclature authority files used for the identification of chemical substances cited in National Library of Medicine (NLM) databases, including the TOXNET system. | |
Record name | (+)-(S)-Fluoxetine hydrochloride | |
Source | EPA DSSTox | |
URL | https://comptox.epa.gov/dashboard/DTXSID00872289 | |
Description | DSSTox provides a high quality public chemistry resource for supporting improved predictive toxicology. | |
Record name | S-(+)-Fluoxetine hydrochloride | |
Source | European Chemicals Agency (ECHA) | |
URL | https://echa.europa.eu/information-on-chemicals | |
Description | The European Chemicals Agency (ECHA) is an agency of the European Union which is the driving force among regulatory authorities in implementing the EU's groundbreaking chemicals legislation for the benefit of human health and the environment as well as for innovation and competitiveness. | |
Explanation | Use of the information, documents and data from the ECHA website is subject to the terms and conditions of this Legal Notice, and subject to other binding limitations provided for under applicable law, the information, documents and data made available on the ECHA website may be reproduced, distributed and/or used, totally or in part, for non-commercial purposes provided that ECHA is acknowledged as the source: "Source: European Chemicals Agency, http://echa.europa.eu/". Such acknowledgement must be included in each copy of the material. ECHA permits and encourages organisations and individuals to create links to the ECHA website under the following cumulative conditions: Links can only be made to webpages that provide a link to the Legal Notice page. | |
Record name | FLUOXETINE HYDROCHLORIDE, (S)- | |
Source | FDA Global Substance Registration System (GSRS) | |
URL | https://gsrs.ncats.nih.gov/ginas/app/beta/substances/Z4S3JZC091 | |
Description | The FDA Global Substance Registration System (GSRS) enables the efficient and accurate exchange of information on what substances are in regulated products. Instead of relying on names, which vary across regulatory domains, countries, and regions, the GSRS knowledge base makes it possible for substances to be defined by standardized, scientific descriptions. | |
Explanation | Unless otherwise noted, the contents of the FDA website (www.fda.gov), both text and graphics, are not copyrighted. They are in the public domain and may be republished, reprinted and otherwise used freely by anyone without the need to obtain permission from FDA. Credit to the U.S. Food and Drug Administration as the source is appreciated but not required. | |
Retrosynthesis Analysis
AI-Powered Synthesis Planning: Our tool employs the Template_relevance Pistachio, Template_relevance Bkms_metabolic, Template_relevance Pistachio_ringbreaker, Template_relevance Reaxys, Template_relevance Reaxys_biocatalysis model, leveraging a vast database of chemical reactions to predict feasible synthetic routes.
One-Step Synthesis Focus: Specifically designed for one-step synthesis, it provides concise and direct routes for your target compounds, streamlining the synthesis process.
Accurate Predictions: Utilizing the extensive PISTACHIO, BKMS_METABOLIC, PISTACHIO_RINGBREAKER, REAXYS, REAXYS_BIOCATALYSIS database, our tool offers high-accuracy predictions, reflecting the latest in chemical research and data.
Strategy Settings
Precursor scoring | Relevance Heuristic |
---|---|
Min. plausibility | 0.01 |
Model | Template_relevance |
Template Set | Pistachio/Bkms_metabolic/Pistachio_ringbreaker/Reaxys/Reaxys_biocatalysis |
Top-N result to add to graph | 6 |
Feasible Synthetic Routes
Avertissement et informations sur les produits de recherche in vitro
Veuillez noter que tous les articles et informations sur les produits présentés sur BenchChem sont destinés uniquement à des fins informatives. Les produits disponibles à l'achat sur BenchChem sont spécifiquement conçus pour des études in vitro, qui sont réalisées en dehors des organismes vivants. Les études in vitro, dérivées du terme latin "in verre", impliquent des expériences réalisées dans des environnements de laboratoire contrôlés à l'aide de cellules ou de tissus. Il est important de noter que ces produits ne sont pas classés comme médicaments et n'ont pas reçu l'approbation de la FDA pour la prévention, le traitement ou la guérison de toute condition médicale, affection ou maladie. Nous devons souligner que toute forme d'introduction corporelle de ces produits chez les humains ou les animaux est strictement interdite par la loi. Il est essentiel de respecter ces directives pour assurer la conformité aux normes légales et éthiques en matière de recherche et d'expérimentation.