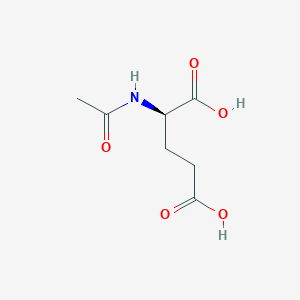
N-Acetyl-D-glutamic acid
Vue d'ensemble
Description
N-Acetyl-D-glutamic acid (NADGA) is a chiral, acetylated derivative of D-glutamic acid. Its chemical formula is C₇H₁₁NO₅, with a molecular weight of 189.17 g/mol . Structurally, it features an acetyl group attached to the amino group of D-glutamic acid, distinguishing it from non-acetylated or L-enantiomeric forms. Physically, NADGA is a white to off-white crystalline solid with a melting point of 192–194°C and a density of 1.354 g/cm³ .
NADGA is primarily utilized in biochemical research, particularly in studies involving microbial metabolism and enzymatic specificity.
Méthodes De Préparation
Synthetic Routes and Reaction Conditions
N-Acetyl-D-glutamic acid can be synthesized through several methods. One common approach involves the reaction of glutamic acid with acetic anhydride in the presence of a base such as pyridine. The reaction typically occurs at room temperature and yields this compound as a white crystalline solid .
Industrial Production Methods
In industrial settings, this compound is often produced using enzymatic methods. The enzyme N-acetylglutamate synthase catalyzes the reaction between glutamic acid and acetyl-CoA to form this compound. This method is preferred due to its high specificity and efficiency .
Analyse Des Réactions Chimiques
Types of Reactions
N-Acetyl-D-glutamic acid undergoes various chemical reactions, including:
Hydrolysis: The acetyl group can be hydrolyzed to yield glutamic acid.
Oxidation: The compound can be oxidized to form corresponding oxo derivatives.
Substitution: The acetyl group can be substituted with other functional groups under specific conditions.
Common Reagents and Conditions
Hydrolysis: Typically performed in acidic or basic conditions using water as the reagent.
Oxidation: Common oxidizing agents include potassium permanganate and hydrogen peroxide.
Substitution: Various nucleophiles can be used to replace the acetyl group, often requiring catalysts or specific reaction conditions.
Major Products Formed
Hydrolysis: Glutamic acid
Oxidation: Oxo derivatives of this compound
Substitution: Derivatives with different functional groups replacing the acetyl group
Applications De Recherche Scientifique
N-Acetyl-D-glutamic acid has a wide range of applications in scientific research:
Chemistry: Used as a precursor in the synthesis of various organic compounds.
Biology: Plays a role in the study of metabolic pathways, particularly in the urea cycle and arginine biosynthesis.
Medicine: Investigated for its potential therapeutic effects in treating metabolic disorders related to the urea cycle.
Industry: Utilized in the production of flavor enhancers and food additives
Mécanisme D'action
N-Acetyl-D-glutamic acid exerts its effects primarily through its role as an allosteric activator of carbamoyl phosphate synthetase I, an enzyme involved in the urea cycle. By activating this enzyme, this compound enhances the conversion of ammonia to urea, thereby reducing ammonia toxicity in the body. This regulatory mechanism is crucial for maintaining nitrogen balance and preventing hyperammonemia .
Comparaison Avec Des Composés Similaires
Structural Analogs
N-Acetyl-L-Glutamic Acid (NALGA)
- Molecular Weight : 189.17 g/mol (identical to NADGA).
- Key Difference : Enantiomeric configuration (L-form vs. D-form).
- Biological Role : Essential in the urea cycle as a cofactor for carbamoyl phosphate synthetase I (CPS I), facilitating ammonia detoxification in mammals .
- Metabolic Activity: Substrate for Pseudomonas aeruginosa N-acetylglutamate deacetylase, enabling bacterial growth .
N-Acetyl-D-Aspartic Acid (NADAA)
- Molecular Formula: C₆H₉NO₅ (vs. C₇H₁₁NO₅ for NADGA).
- Molecular Weight : 175.1 g/mol .
- Structural Difference : Shorter carbon backbone (aspartic acid has four carbons vs. glutamic acid’s five).
- Functional Role : Found in neural tissue; implicated in neurotransmitter synthesis .
N-Propionyl-L-Glutamic Acid (NPLGA)
- Molecular Formula: C₈H₁₃NO₅ (propionyl group replaces acetyl).
- Functional Role : Acts as an alternative cofactor in CPS I activation but with lower efficacy than NALGA .
Physicochemical Properties
Key Observations :
- Enantiomeric Differences : Despite identical molecular weights, NADGA and NALGA differ in solubility and biological activity due to stereochemical preferences in enzymes .
- Backbone Length : NADGA (5-carbon backbone) is less water-soluble than NADAA (4-carbon), likely due to increased hydrophobic interactions .
This compound
- Research Use: Probe for studying bacterial deacetylases (e.g., in P. aeruginosa) due to its resistance to hydrolysis in eukaryotic systems .
- Downstream Applications : Precursor for synthesizing derivatives like L-carnitine .
N-Acetyl-L-Glutamic Acid
- Clinical Relevance : Used to treat hyperammonemia by enhancing CPS I activity .
- Agricultural Use: Precursor for nodulation factors in legume-rhizobia symbiosis .
N-Acetyl-D-Glucosamine (NAG)
- Molecular Formula: C₈H₁₅NO₆ (distinct from NADGA).
- Role : Component of bacterial cell walls and chitin; used in osteoarthritis supplements .
Research Findings and Enzymatic Specificity
- Enzyme Substrate Preferences: P. aeruginosa N-acetylglutamate deacetylase hydrolyzes NALGA and NPLGA but shows negligible activity toward NADGA . Mammalian CPS I exclusively recognizes the L-enantiomer, highlighting evolutionary conservation of stereochemical specificity .
Synthetic Utility :
- NADGA’s stability in aqueous solutions makes it a candidate for designing enzyme-stable analogs in drug delivery .
Activité Biologique
N-Acetyl-D-glutamic acid (NAG) is a derivative of glutamic acid, notable for its role in various biological processes, particularly in the urea cycle and amino acid metabolism. This article provides a comprehensive overview of the biological activities associated with this compound, supported by research findings, case studies, and data tables.
1. Biosynthesis and Metabolism
This compound is synthesized primarily from glutamate and acetyl-CoA through the action of the enzyme N-acetylglutamate synthase (NAGS). This compound serves as a critical allosteric activator of mitochondrial carbamyl phosphate synthetase I (CPSI), the first enzyme in the urea cycle. The presence of NAG is essential for CPSI activation, which facilitates the conversion of ammonia into urea for excretion, thereby playing a crucial role in nitrogen metabolism in vertebrates .
Table 1: Key Enzymatic Reactions Involving this compound
Enzyme | Reaction | Function |
---|---|---|
N-acetylglutamate synthase (NAGS) | Glutamate + Acetyl-CoA → this compound | Synthesis of NAG |
Carbamyl phosphate synthetase I (CPSI) | This compound + NH₃ + CO₂ → Carbamyl phosphate | Initiation of urea cycle |
2.1 Urea Cycle Regulation
This compound plays a pivotal role in regulating the urea cycle, particularly in conditions where ammonia levels are elevated. Its function as an allosteric activator for CPSI underscores its importance in maintaining nitrogen balance and preventing hyperammonemia, a condition that can lead to severe neurological impairment if untreated .
2.2 Role in Arginine Biosynthesis
In addition to its role in the urea cycle, NAG is also involved as an intermediate in arginine biosynthesis. This pathway is vital for protein synthesis and various metabolic functions, including the production of nitric oxide, which is important for vascular health .
3.1 NAGS Deficiency
A notable clinical condition related to this compound is N-acetylglutamate synthase (NAGS) deficiency, which is characterized by life-threatening hyperammonemia due to impaired urea cycle function. Early diagnosis and treatment with carbaglumic acid (a synthetic analogue of NAG) have shown promising outcomes in managing this condition. Case studies indicate that patients receiving timely intervention demonstrate normal neurological development despite initial severe hyperammonemia .
Case Study Example:
- A newborn diagnosed with severe hyperammonemia was treated with carbaglumic acid shortly after birth. The patient exhibited normal growth and neurological development at follow-up examinations .
4. Pharmacokinetics and Bioavailability
Recent studies have explored the pharmacokinetics of acetylated amino acids, including this compound. Research indicates that NAG can effectively cross the blood-brain barrier and exhibits favorable bioavailability characteristics when administered .
Table 2: Pharmacokinetic Parameters of this compound
Parameter | Value |
---|---|
C_max (Blood) | Varies with dosage |
Half-life | Approximately 2-4 hours |
Bioavailability | High (>70% under optimal conditions) |
5. Conclusion
This compound is integral to several metabolic pathways, particularly those involved in nitrogen metabolism and amino acid synthesis. Its role as an allosteric activator in the urea cycle highlights its importance in preventing toxic accumulation of ammonia. Furthermore, advancements in understanding its pharmacokinetics may enhance therapeutic strategies for managing metabolic disorders linked to NAGS deficiency.
Q & A
Basic Research Questions
Q. How can N-Acetyl-D-glutamic acid be accurately quantified in complex biological matrices using HPLC?
Methodological Answer: Reverse-phase HPLC coupled with UV/Vis detection (210–220 nm) is recommended for quantification. Optimize the mobile phase (e.g., phosphate buffer pH 2.5 and acetonitrile gradient) to separate the compound from interfering metabolites. Pre-column derivatization with agents like 6-mercapto-1-hexanol (MCH) or 11-mercaptoundecanoic acid (MUA) enhances detection sensitivity. Validate the method using standard curves (0.1–100 µM) with a retention time of 8–10 minutes under isocratic conditions .
Table 1: HPLC Parameters for Quantification
Parameter | Value |
---|---|
Column | C18 (5 µm, 250 × 4.6 mm) |
Mobile Phase | 10 mM phosphate buffer (pH 2.5):acetonitrile (90:10) |
Flow Rate | 1.0 mL/min |
Detection Wavelength | 210 nm |
Retention Time | ~8.5 minutes |
Q. What are the standard protocols for synthesizing this compound in laboratory settings?
Methodological Answer: Synthesize the compound via acetylation of D-glutamic acid using acetic anhydride in an alkaline medium (pH 8–9). Purify the product via recrystallization from ethanol/water (1:3 v/v) and confirm purity (>98%) using melting point analysis (192–194°C) and FTIR (characteristic peaks at 1650 cm⁻¹ for amide C=O and 1540 cm⁻¹ for N-H bend) .
Advanced Research Questions
Q. How do solvent-mediated polymorphic transformations of this compound affect its crystallographic properties, and what in situ techniques can monitor these changes?
Methodological Answer: Polymorphic transitions (e.g., α ↔ β forms) are influenced by solvent polarity and temperature. Use Raman spectroscopy (shift at 1720 cm⁻¹ for carbonyl groups) to track crystal lattice rearrangements and ATR-FTIR (amide I/II bands) to monitor liquid-phase concentration dynamics. Combine with focused beam reflectance measurement (FBRM) to analyze particle size distribution during crystallization. Model nucleation/growth kinetics using population balance equations .
Q. What is the role of this compound in regulating the urea cycle, and how can kinetic parameters of related enzymes be determined?
Methodological Answer: this compound is a cofactor for carbamoyl phosphate synthetase I (CPS I) , activating ammonia incorporation into the urea cycle. To measure CPS I kinetics:
- Prepare mitochondrial extracts from liver tissue.
- Use a spectrophotometric assay (340 nm) to track NADH consumption linked to carbamoyl phosphate production.
- Calculate (2–5 mM) and using varying acetylglutamate concentrations (0–10 mM). Reference hyperammonemia models to validate regulatory disruptions .
Q. How can chiral resolution agents like this compound be utilized in the synthesis of enantiomerically pure compounds such as L-carnitine?
Methodological Answer: this compound acts as a chiral selector in diastereomeric salt formation. For L-carnitine synthesis:
- React racemic D,L-carnitine with this compound in ethanol.
- Isolate the L-carnitine diastereomer via fractional crystallization (yield >85%).
- Confirm enantiomeric excess (>99%) using chiral HPLC (e.g., Chirobiotic T column) .
Table 2: Optical Resolution Conditions for L-Carnitine
Parameter | Value |
---|---|
Solvent | Ethanol |
Molar Ratio | 1:1 (carnitine:acetylglutamate) |
Temperature | 25°C |
Crystallization Yield | 85–90% |
Q. Data Contradiction Analysis
Q. How can discrepancies in reported solubility profiles of this compound across studies be resolved?
Methodological Answer: Solubility variations (e.g., in DMSO vs. water) arise from differences in pH, ionic strength, and measurement techniques. Standardize protocols by:
- Using saturated solutions equilibrated at 25°C for 24 hours.
- Quantifying solubility via gravimetric analysis or UV absorbance.
- Cross-validating with NIST reference data (e.g., molecular weight 189.17 g/mol) to ensure consistency .
Q. Experimental Design Considerations
Q. What strategies optimize the stability of this compound in long-term enzymatic assays?
Methodological Answer:
Propriétés
IUPAC Name |
(2R)-2-acetamidopentanedioic acid | |
---|---|---|
Source | PubChem | |
URL | https://pubchem.ncbi.nlm.nih.gov | |
Description | Data deposited in or computed by PubChem | |
InChI |
InChI=1S/C7H11NO5/c1-4(9)8-5(7(12)13)2-3-6(10)11/h5H,2-3H2,1H3,(H,8,9)(H,10,11)(H,12,13)/t5-/m1/s1 | |
Source | PubChem | |
URL | https://pubchem.ncbi.nlm.nih.gov | |
Description | Data deposited in or computed by PubChem | |
InChI Key |
RFMMMVDNIPUKGG-RXMQYKEDSA-N | |
Source | PubChem | |
URL | https://pubchem.ncbi.nlm.nih.gov | |
Description | Data deposited in or computed by PubChem | |
Canonical SMILES |
CC(=O)NC(CCC(=O)O)C(=O)O | |
Source | PubChem | |
URL | https://pubchem.ncbi.nlm.nih.gov | |
Description | Data deposited in or computed by PubChem | |
Isomeric SMILES |
CC(=O)N[C@H](CCC(=O)O)C(=O)O | |
Source | PubChem | |
URL | https://pubchem.ncbi.nlm.nih.gov | |
Description | Data deposited in or computed by PubChem | |
Molecular Formula |
C7H11NO5 | |
Source | PubChem | |
URL | https://pubchem.ncbi.nlm.nih.gov | |
Description | Data deposited in or computed by PubChem | |
DSSTOX Substance ID |
DTXSID70364404 | |
Record name | N-ACETYL-D-GLUTAMIC ACID | |
Source | EPA DSSTox | |
URL | https://comptox.epa.gov/dashboard/DTXSID70364404 | |
Description | DSSTox provides a high quality public chemistry resource for supporting improved predictive toxicology. | |
Molecular Weight |
189.17 g/mol | |
Source | PubChem | |
URL | https://pubchem.ncbi.nlm.nih.gov | |
Description | Data deposited in or computed by PubChem | |
CAS No. |
5817-08-3, 19146-55-5 | |
Record name | Glutamic acid, N-acetyl- | |
Source | EPA Chemicals under the TSCA | |
URL | https://www.epa.gov/chemicals-under-tsca | |
Description | EPA Chemicals under the Toxic Substances Control Act (TSCA) collection contains information on chemicals and their regulations under TSCA, including non-confidential content from the TSCA Chemical Substance Inventory and Chemical Data Reporting. | |
Record name | N-ACETYL-D-GLUTAMIC ACID | |
Source | EPA DSSTox | |
URL | https://comptox.epa.gov/dashboard/DTXSID70364404 | |
Description | DSSTox provides a high quality public chemistry resource for supporting improved predictive toxicology. | |
Retrosynthesis Analysis
AI-Powered Synthesis Planning: Our tool employs the Template_relevance Pistachio, Template_relevance Bkms_metabolic, Template_relevance Pistachio_ringbreaker, Template_relevance Reaxys, Template_relevance Reaxys_biocatalysis model, leveraging a vast database of chemical reactions to predict feasible synthetic routes.
One-Step Synthesis Focus: Specifically designed for one-step synthesis, it provides concise and direct routes for your target compounds, streamlining the synthesis process.
Accurate Predictions: Utilizing the extensive PISTACHIO, BKMS_METABOLIC, PISTACHIO_RINGBREAKER, REAXYS, REAXYS_BIOCATALYSIS database, our tool offers high-accuracy predictions, reflecting the latest in chemical research and data.
Strategy Settings
Precursor scoring | Relevance Heuristic |
---|---|
Min. plausibility | 0.01 |
Model | Template_relevance |
Template Set | Pistachio/Bkms_metabolic/Pistachio_ringbreaker/Reaxys/Reaxys_biocatalysis |
Top-N result to add to graph | 6 |
Feasible Synthetic Routes
Avertissement et informations sur les produits de recherche in vitro
Veuillez noter que tous les articles et informations sur les produits présentés sur BenchChem sont destinés uniquement à des fins informatives. Les produits disponibles à l'achat sur BenchChem sont spécifiquement conçus pour des études in vitro, qui sont réalisées en dehors des organismes vivants. Les études in vitro, dérivées du terme latin "in verre", impliquent des expériences réalisées dans des environnements de laboratoire contrôlés à l'aide de cellules ou de tissus. Il est important de noter que ces produits ne sont pas classés comme médicaments et n'ont pas reçu l'approbation de la FDA pour la prévention, le traitement ou la guérison de toute condition médicale, affection ou maladie. Nous devons souligner que toute forme d'introduction corporelle de ces produits chez les humains ou les animaux est strictement interdite par la loi. Il est essentiel de respecter ces directives pour assurer la conformité aux normes légales et éthiques en matière de recherche et d'expérimentation.