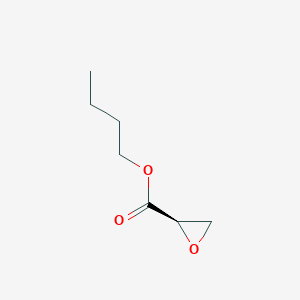
Butyl (2R)-oxirane-2-carboxylate
- Cliquez sur DEMANDE RAPIDE pour recevoir un devis de notre équipe d'experts.
- Avec des produits de qualité à un prix COMPÉTITIF, vous pouvez vous concentrer davantage sur votre recherche.
Vue d'ensemble
Description
The description of a chemical compound usually includes its IUPAC name, molecular formula, and structure. It may also include its appearance and odor .
Synthesis Analysis
The synthesis of a compound involves the processes used to create it. This could involve various chemical reactions, the use of catalysts, and specific conditions such as temperature and pressure .Molecular Structure Analysis
This involves understanding the arrangement of atoms in the molecule and the type of bonds between them. Tools like X-ray crystallography, NMR spectroscopy, and computational chemistry are often used .Chemical Reactions Analysis
This involves studying how the compound reacts with other substances. It includes understanding the reaction mechanism, the products formed, and the conditions required for the reaction .Physical And Chemical Properties Analysis
This includes studying properties like melting point, boiling point, solubility, density, and reactivity .Mécanisme D'action
Safety and Hazards
Orientations Futures
Propriétés
IUPAC Name |
butyl (2R)-oxirane-2-carboxylate |
Source
|
---|---|---|
Source | PubChem | |
URL | https://pubchem.ncbi.nlm.nih.gov | |
Description | Data deposited in or computed by PubChem | |
InChI |
InChI=1S/C7H12O3/c1-2-3-4-9-7(8)6-5-10-6/h6H,2-5H2,1H3/t6-/m1/s1 |
Source
|
Source | PubChem | |
URL | https://pubchem.ncbi.nlm.nih.gov | |
Description | Data deposited in or computed by PubChem | |
InChI Key |
PDIRPVPNNLOAKW-ZCFIWIBFSA-N |
Source
|
Source | PubChem | |
URL | https://pubchem.ncbi.nlm.nih.gov | |
Description | Data deposited in or computed by PubChem | |
Canonical SMILES |
CCCCOC(=O)C1CO1 |
Source
|
Source | PubChem | |
URL | https://pubchem.ncbi.nlm.nih.gov | |
Description | Data deposited in or computed by PubChem | |
Isomeric SMILES |
CCCCOC(=O)[C@H]1CO1 |
Source
|
Source | PubChem | |
URL | https://pubchem.ncbi.nlm.nih.gov | |
Description | Data deposited in or computed by PubChem | |
Molecular Formula |
C7H12O3 |
Source
|
Source | PubChem | |
URL | https://pubchem.ncbi.nlm.nih.gov | |
Description | Data deposited in or computed by PubChem | |
Molecular Weight |
144.17 g/mol |
Source
|
Source | PubChem | |
URL | https://pubchem.ncbi.nlm.nih.gov | |
Description | Data deposited in or computed by PubChem | |
Product Name |
Butyl (2R)-oxirane-2-carboxylate |
Avertissement et informations sur les produits de recherche in vitro
Veuillez noter que tous les articles et informations sur les produits présentés sur BenchChem sont destinés uniquement à des fins informatives. Les produits disponibles à l'achat sur BenchChem sont spécifiquement conçus pour des études in vitro, qui sont réalisées en dehors des organismes vivants. Les études in vitro, dérivées du terme latin "in verre", impliquent des expériences réalisées dans des environnements de laboratoire contrôlés à l'aide de cellules ou de tissus. Il est important de noter que ces produits ne sont pas classés comme médicaments et n'ont pas reçu l'approbation de la FDA pour la prévention, le traitement ou la guérison de toute condition médicale, affection ou maladie. Nous devons souligner que toute forme d'introduction corporelle de ces produits chez les humains ou les animaux est strictement interdite par la loi. Il est essentiel de respecter ces directives pour assurer la conformité aux normes légales et éthiques en matière de recherche et d'expérimentation.