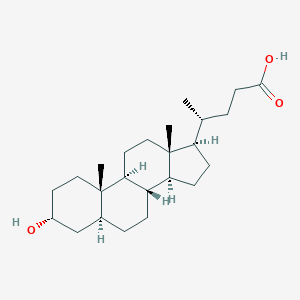
Acide allolithocholique
Vue d'ensemble
Description
L’acide allolithocholique est un acide biliaire, plus précisément un acide biliaire secondaire, que l’on retrouve dans le sérum et les fèces normaux. Il est connu pour son rôle dans la promotion de l’excrétion, de l’absorption et du transport des graisses et des stérols dans les intestins et le foie . L’this compound est également reconnu pour sa présence en concentrations plus élevées chez les patients atteints d’un cancer colorectal .
Applications De Recherche Scientifique
Immunological Applications
Allolithocholic acid has been identified as a modulator of regulatory T cells (Tregs). Research indicates that alloLCA increases levels of Foxp3, a transcription factor crucial for Treg differentiation. This modulation suggests potential therapeutic benefits in managing autoimmune disorders where Treg function is compromised .
Case Study: Autoimmune Disorders
- Background : Autoimmune diseases often involve dysregulated immune responses.
- Findings : In vitro studies have shown that alloLCA can enhance the differentiation of Tregs, potentially improving immune tolerance in conditions like multiple sclerosis and rheumatoid arthritis .
Metabolic Regulation
Table 1: Effects of Allolithocholic Acid on Metabolic Pathways
Application | Mechanism | Outcome |
---|---|---|
Activation of BK channels | Modulation of calcium signaling | Improved vascular function |
Regulation of bile flow | Reduces hepatic bile flow and cholesterol secretion | Potential treatment for cholestasis |
Influence on gut microbiota | Alters bile acid composition | May impact metabolic diseases |
Therapeutic Potential in Cancer
Case Study: Colon Cancer
- Background : The relationship between bile acids and colorectal cancer has been extensively studied.
- Findings : Increased alloLCA levels correlate with tumor progression, indicating its potential as a diagnostic marker or therapeutic target .
Synthesis and Accessibility Challenges
Despite its promising applications, allolithocholic acid is not commercially available, necessitating synthetic approaches for research purposes. Recent studies have developed stereoselective synthesis methods to produce alloLCA efficiently, which is crucial for advancing its application in clinical research .
Table 2: Synthesis Overview of Allolithocholic Acid
Synthesis Step | Description | Yield (%) |
---|---|---|
Protection of C(24) carboxylic acid | Initial step to prepare for oxidation | Not specified |
Oxidation to unsaturated ketone | Key transformation using PCC and HIO3 | Not specified |
Stereoselective reduction | Accomplished using copper hydride conditions | 27% |
Mécanisme D'action
Le mécanisme d’action de l’acide allolithocholique implique son interaction avec des cibles moléculaires et des voies spécifiques. L’une des cibles clés est les canaux potassiques activés par le calcium à grande conductance (BK/KCa), qui sont activés par l’this compound . Cette activation entraîne divers effets en aval, notamment des changements dans l’équilibre ionique cellulaire et les voies de signalisation. De plus, il a été démontré que l’this compound se lie avec une forte affinité à l’enzyme membranaire humaine NAPE-PLD, ce qui améliore l’assemblage des dimères et permet la catalyse .
Analyse Biochimique
Biochemical Properties
Allolithocholic acid interacts with various biomolecules to facilitate the excretion, absorption, and transport of fats and sterols in the intestine and liver . It is involved in the metabolism of lipids, particularly fats and sterols
Cellular Effects
Allolithocholic acid has been found to influence cell function, particularly in the intestine and liver where it facilitates the excretion, absorption, and transport of fats and sterols . It is also suggested to have effects on cell signaling pathways, gene expression, and cellular metabolism
Molecular Mechanism
It is known to facilitate the excretion, absorption, and transport of fats and sterols in the intestine and liver This suggests that it may interact with biomolecules involved in these processes, potentially influencing enzyme activity and gene expression
Temporal Effects in Laboratory Settings
It is known that Allolithocholic acid is a stable compound , but information on its degradation and long-term effects on cellular function in in vitro or in vivo studies is limited.
Metabolic Pathways
Allolithocholic acid is involved in the metabolism of lipids, particularly fats and sterols . It facilitates their excretion, absorption, and transport in the intestine and liver
Transport and Distribution
Allolithocholic acid is known to facilitate the excretion, absorption, and transport of fats and sterols in the intestine and liver This suggests that it may interact with transporters or binding proteins involved in these processes
Subcellular Localization
Given its role in facilitating the excretion, absorption, and transport of fats and sterols in the intestine and liver , it is likely that it localizes to areas of the cell involved in these processes
Méthodes De Préparation
L’acide allolithocholique peut être synthétisé par différentes méthodes. Une voie de synthèse courante implique l’épimérisation de l’hydrogène C-5 dans les dérivés de l’acide cholique . Ce processus nécessite généralement des conditions de réaction spécifiques, telles que l’utilisation de bases ou d’acides forts, pour faciliter l’épimérisation. Les méthodes de production industrielle impliquent souvent l’extraction et la purification de l’this compound à partir de sources naturelles, telles que la bile, suivie d’une modification chimique pour obtenir le composé souhaité .
Analyse Des Réactions Chimiques
L’acide allolithocholique subit plusieurs types de réactions chimiques, notamment :
Réduction : Cette réaction implique l’élimination de l’oxygène ou l’addition d’hydrogène, généralement à l’aide de réactifs tels que l’hydrure de lithium et d’aluminium ou le borohydrure de sodium.
Substitution : Cette réaction implique le remplacement d’un groupe fonctionnel par un autre, souvent à l’aide de réactifs tels que les halogènes ou les nucléophiles.
Les réactifs et les conditions courants utilisés dans ces réactions comprennent les acides ou les bases forts, les agents oxydants et les agents réducteurs. Les principaux produits formés à partir de ces réactions dépendent des conditions et des réactifs spécifiques utilisés, mais ils comprennent souvent des acides biliaires modifiés avec des groupes fonctionnels différents .
Applications de la recherche scientifique
L’this compound a une large gamme d’applications de recherche scientifique, notamment :
Chimie : Il est utilisé comme composé modèle pour étudier le comportement des acides biliaires et de leurs dérivés.
Biologie : Il est utilisé pour étudier le rôle des acides biliaires dans divers processus biologiques, tels que le métabolisme des graisses et l’homéostasie du cholestérol.
Industrie : Il est utilisé dans la production de divers produits pharmaceutiques et comme outil de recherche dans le développement de nouveaux médicaments
Comparaison Avec Des Composés Similaires
L’acide allolithocholique est similaire aux autres acides biliaires, tels que l’acide lithocholique et l’acide cholique. Il est unique à plusieurs égards :
Acide lithocholique : Les deux sont des acides biliaires secondaires, mais l’this compound a une stéréochimie différente au niveau de la position C-5, ce qui affecte son activité biologique.
D’autres composés similaires comprennent l’acide déhydrolithocholique, l’acide isolithocholique et l’acide tauroallolithocholanoïque .
Activité Biologique
Allolithocholic acid (allo-LCA) is a secondary bile acid that has garnered attention due to its unique biological activities and potential therapeutic applications. This article delves into the biological activity of allo-LCA, including its formation, mechanisms of action, and implications for health and disease, particularly in relation to gut microbiota and metabolic disorders.
Formation and Sources
Allolithocholic acid is derived from lithocholic acid through microbial biotransformation in the gut. The gut microbiome plays a crucial role in the conversion of primary bile acids into their secondary forms. Specifically, Firmicutes bacteria are known to facilitate this transformation through enzymes that catalyze the reduction of lithocholic acid to allo-LCA. Recent studies have identified specific genes such as baiA1 and baiP that are responsible for this conversion, highlighting the intricate relationship between gut microbiota and bile acid metabolism .
The biological activity of allo-LCA is multifaceted, influencing various physiological processes:
- Antimicrobial Activity : Allo-LCA exhibits significant antimicrobial properties, particularly against pathogenic bacteria such as Clostridioides difficile. It has been shown to inhibit the growth and toxin production of this pathogen while sparing beneficial gut microbiota . This selective action suggests its potential as a therapeutic agent in managing gastrointestinal infections.
- Anti-inflammatory Effects : Allo-LCA has demonstrated anti-inflammatory properties by modulating immune responses. It influences T cell differentiation and reduces inflammatory cytokine production, which could be beneficial in conditions characterized by chronic inflammation such as inflammatory bowel disease (IBD) .
- Gut Barrier Function : Research indicates that allo-LCA may enhance the integrity of the intestinal barrier. By promoting tight junction formation between epithelial cells, it helps maintain gut homeostasis and prevent conditions like leaky gut syndrome .
Case Studies and Research Findings
Recent studies have provided insights into the clinical relevance of allo-LCA:
- Colorectal Cancer : Increased levels of allo-LCA have been observed in fecal samples from colorectal cancer patients compared to healthy individuals. This correlation suggests that allo-LCA might serve as a biomarker for disease progression or a target for therapeutic intervention .
- Metabolic Disorders : In metabolic syndrome models, allo-LCA has been linked to improved lipid metabolism and reduced hepatic steatosis. Its role in regulating bile acid signaling pathways may contribute to these beneficial effects .
- Gut Microbiota Modulation : Dietary interventions that promote the growth of Firmicutes have been shown to increase allo-LCA levels, thereby enhancing its protective effects against pathogenic bacteria and supporting overall gut health .
Comparative Analysis of Bile Acids
The following table summarizes key differences between lithocholic acid (LCA) and its epimer, allo-LCA:
Property | Lithocholic Acid (LCA) | Allolithocholic Acid (allo-LCA) |
---|---|---|
Structure | Kinked | Flat |
Antimicrobial Activity | Moderate | Strong against C. difficile |
Anti-inflammatory Activity | Limited | Significant |
Gut Barrier Protection | Minimal | Enhanced |
Metabolic Effects | Potentially harmful | Beneficial |
Propriétés
IUPAC Name |
(4R)-4-[(3R,5S,8R,9S,10S,13R,14S,17R)-3-hydroxy-10,13-dimethyl-2,3,4,5,6,7,8,9,11,12,14,15,16,17-tetradecahydro-1H-cyclopenta[a]phenanthren-17-yl]pentanoic acid | |
---|---|---|
Source | PubChem | |
URL | https://pubchem.ncbi.nlm.nih.gov | |
Description | Data deposited in or computed by PubChem | |
InChI |
InChI=1S/C24H40O3/c1-15(4-9-22(26)27)19-7-8-20-18-6-5-16-14-17(25)10-12-23(16,2)21(18)11-13-24(19,20)3/h15-21,25H,4-14H2,1-3H3,(H,26,27)/t15-,16+,17-,18+,19-,20+,21+,23+,24-/m1/s1 | |
Source | PubChem | |
URL | https://pubchem.ncbi.nlm.nih.gov | |
Description | Data deposited in or computed by PubChem | |
InChI Key |
SMEROWZSTRWXGI-NWFSOSCSSA-N | |
Source | PubChem | |
URL | https://pubchem.ncbi.nlm.nih.gov | |
Description | Data deposited in or computed by PubChem | |
Canonical SMILES |
CC(CCC(=O)O)C1CCC2C1(CCC3C2CCC4C3(CCC(C4)O)C)C | |
Source | PubChem | |
URL | https://pubchem.ncbi.nlm.nih.gov | |
Description | Data deposited in or computed by PubChem | |
Isomeric SMILES |
C[C@H](CCC(=O)O)[C@H]1CC[C@@H]2[C@@]1(CC[C@H]3[C@H]2CC[C@@H]4[C@@]3(CC[C@H](C4)O)C)C | |
Source | PubChem | |
URL | https://pubchem.ncbi.nlm.nih.gov | |
Description | Data deposited in or computed by PubChem | |
Molecular Formula |
C24H40O3 | |
Source | PubChem | |
URL | https://pubchem.ncbi.nlm.nih.gov | |
Description | Data deposited in or computed by PubChem | |
DSSTOX Substance ID |
DTXSID90415285 | |
Record name | AC1NR32F | |
Source | EPA DSSTox | |
URL | https://comptox.epa.gov/dashboard/DTXSID90415285 | |
Description | DSSTox provides a high quality public chemistry resource for supporting improved predictive toxicology. | |
Molecular Weight |
376.6 g/mol | |
Source | PubChem | |
URL | https://pubchem.ncbi.nlm.nih.gov | |
Description | Data deposited in or computed by PubChem | |
CAS No. |
2276-94-0 | |
Record name | (3α,5α)-3-Hydroxycholan-24-oic acid | |
Source | CAS Common Chemistry | |
URL | https://commonchemistry.cas.org/detail?cas_rn=2276-94-0 | |
Description | CAS Common Chemistry is an open community resource for accessing chemical information. Nearly 500,000 chemical substances from CAS REGISTRY cover areas of community interest, including common and frequently regulated chemicals, and those relevant to high school and undergraduate chemistry classes. This chemical information, curated by our expert scientists, is provided in alignment with our mission as a division of the American Chemical Society. | |
Explanation | The data from CAS Common Chemistry is provided under a CC-BY-NC 4.0 license, unless otherwise stated. | |
Record name | AC1NR32F | |
Source | EPA DSSTox | |
URL | https://comptox.epa.gov/dashboard/DTXSID90415285 | |
Description | DSSTox provides a high quality public chemistry resource for supporting improved predictive toxicology. | |
Retrosynthesis Analysis
AI-Powered Synthesis Planning: Our tool employs the Template_relevance Pistachio, Template_relevance Bkms_metabolic, Template_relevance Pistachio_ringbreaker, Template_relevance Reaxys, Template_relevance Reaxys_biocatalysis model, leveraging a vast database of chemical reactions to predict feasible synthetic routes.
One-Step Synthesis Focus: Specifically designed for one-step synthesis, it provides concise and direct routes for your target compounds, streamlining the synthesis process.
Accurate Predictions: Utilizing the extensive PISTACHIO, BKMS_METABOLIC, PISTACHIO_RINGBREAKER, REAXYS, REAXYS_BIOCATALYSIS database, our tool offers high-accuracy predictions, reflecting the latest in chemical research and data.
Strategy Settings
Precursor scoring | Relevance Heuristic |
---|---|
Min. plausibility | 0.01 |
Model | Template_relevance |
Template Set | Pistachio/Bkms_metabolic/Pistachio_ringbreaker/Reaxys/Reaxys_biocatalysis |
Top-N result to add to graph | 6 |
Feasible Synthetic Routes
Q1: How is allolithocholic acid related to cholestatic liver disease?
A1: Research suggests that allolithocholic acid, an unsaturated monohydroxy bile acid, is present in the serum of some patients with cholestatic liver disease. A study examining different bile acid classes found 12 out of 15 patients had conjugated monohydroxy bile acids, including compounds with chromatographic properties similar to taurolithocholic acid and glycolithocholic acid []. Further analysis revealed the presence of a taurine-conjugated unsaturated monohydroxy steroid carboxylic acid with two double bonds, one located in the side chain. While not explicitly identified as allolithocholic acid in this study, the description aligns with its characteristics.
Q2: Can allolithocholic acid serve as a predictor for preeclampsia?
A2: While more research is needed to definitively claim allolithocholic acid as a predictor for preeclampsia, a study investigating metabolomic markers for this condition identified allolithocholic acid as a potential component of the characteristic metabolite profile []. Specifically, it was among a set of third-trimester specific metabolites found in the first-trimester blood plasma of women who later developed preeclampsia. This suggests a possible role for allolithocholic acid in the lipid metabolism disorders and oxidative stress associated with preeclampsia development.
Avertissement et informations sur les produits de recherche in vitro
Veuillez noter que tous les articles et informations sur les produits présentés sur BenchChem sont destinés uniquement à des fins informatives. Les produits disponibles à l'achat sur BenchChem sont spécifiquement conçus pour des études in vitro, qui sont réalisées en dehors des organismes vivants. Les études in vitro, dérivées du terme latin "in verre", impliquent des expériences réalisées dans des environnements de laboratoire contrôlés à l'aide de cellules ou de tissus. Il est important de noter que ces produits ne sont pas classés comme médicaments et n'ont pas reçu l'approbation de la FDA pour la prévention, le traitement ou la guérison de toute condition médicale, affection ou maladie. Nous devons souligner que toute forme d'introduction corporelle de ces produits chez les humains ou les animaux est strictement interdite par la loi. Il est essentiel de respecter ces directives pour assurer la conformité aux normes légales et éthiques en matière de recherche et d'expérimentation.