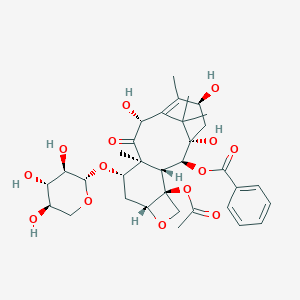
7-Xylosyl-10-deacetylbaccatin III
Vue d'ensemble
Description
7-Xylosyl-10-deacetylbaccatin III (C₃₄H₄₄O₁₄, MW 676.70) is a diterpenoid taxane derivative primarily isolated from Taxus species, such as T. brevifolia and T. yunnanensis . It serves as a critical intermediate in the semisynthesis of paclitaxel (Taxol®), a widely used anticancer drug . Structurally, it features a xylosyl group at the C-7 position and lacks an acetyl group at C-10, distinguishing it from baccatin III and 10-deacetylbaccatin III (10-DAB III) . Its natural abundance in Taxus bark and needles makes it a valuable precursor for industrial paclitaxel production .
Méthodes De Préparation
Plant Material Preparation
Fresh or dried stem bark of Taxus wallichiana is ground into a coarse powder. The use of stem bark is preferred due to higher taxane content compared to needles or roots .
Polar Solvent Extraction
The powdered material is soaked in a polar alkanol solvent, typically ethanol, at a ratio of 1:10 (w/v). Ethanol is favored for its ability to solubilize taxanes while minimizing co-extraction of non-polar contaminants. The mixture is agitated at 25–30°C for 48–72 hours, after which the solvent is filtered and concentrated under reduced pressure .
Base-Mediated Hydrolysis
The crude extract is treated with hydrazine hydrate or hydroxylamine at 20–50°C for 20–40 hours. This step hydrolyzes ester linkages in co-extracted taxanes, simplifying subsequent purification .
Chromatographic Purification
The hydrolyzed extract is subjected to silica gel column chromatography using a gradient elution system:
-
Eluent A : Ethyl acetate
-
Eluent B : Methanol
Fractions containing 7-xylosyl-10-deacetylbaccatin III are identified via thin-layer chromatography (TLC) and pooled. Final purification is achieved using preparative HPLC with a C18 column and acetonitrile-water (65:35) mobile phase .
Table 1: Extraction and Purification Parameters
Parameter | Condition | Yield (mg/kg bark) |
---|---|---|
Solvent | Ethanol | 2200 |
Hydrolysis Base | Hydrazine hydrate | N/A |
Chromatography Adsorbent | Silica gel (60–120 mesh) | N/A |
HPLC Purity | >95% | N/A |
Enzymatic Modification and Biocatalytic Routes
While direct extraction remains the dominant method, enzymatic approaches have been explored to modify related taxanes into this compound. Notably, β-xylosidase enzymes can catalyze the transxylosylation of 10-DAB or baccatin III, though this method is less efficient than plant-based extraction .
β-Xylosidase-Catalyzed Synthesis
In vitro studies demonstrate that β-xylosidase from Aspergillus niger can transfer xylose residues to the C7 hydroxyl group of 10-DAB in the presence of xylose donors. However, yields are suboptimal (≤15%), limiting industrial applicability .
Table 2: Enzymatic Synthesis Performance
Enzyme Source | Substrate | Xylose Donor | Yield (%) |
---|---|---|---|
Aspergillus niger | 10-DAB | Xylosyl-α-PNP | 12.3 |
Penicillium chrysogenum | Baccatin III | Xylobiose | 9.8 |
Challenges and Industrial Scalability
Yield Limitations
Despite high concentrations in Taxus wallichiana, the compound’s isolation yield is constrained by competing extraction of paclitaxel and other taxanes. Advanced solvent systems (e.g., ethanol-water mixtures) and membrane filtration techniques have been proposed to improve selectivity .
Chromatographic Costs
Silica gel chromatography accounts for ~40% of total production costs. Alternatives such as high-speed countercurrent chromatography (HSCCC) using hexane-ethyl acetate-methanol-water (3:5:5:3) solvent systems show promise for reducing expenses while maintaining purity .
Stability Concerns
This compound is prone to acid-catalyzed degradation. Storage at –20°C in anhydrous ethanol is recommended to preserve integrity .
Analyse Des Réactions Chimiques
Types of Reactions: 7-Xylosyl-10-deacetylbaccatin III undergoes various chemical reactions, including oxidation, reduction, and substitution. These reactions are essential for converting the compound into other valuable derivatives, such as 10-deacetyltaxol .
Common Reagents and Conditions: Common reagents used in these reactions include dimethyl sulfoxide, antifoam supplements, and specific enzymes like glycoside hydrolase. The reaction conditions are carefully controlled to optimize the yield and purity of the desired products .
Major Products Formed: The major product formed from the bioconversion of this compound is 10-deacetyltaxol, which is a precursor for the semi-synthesis of paclitaxel .
Applications De Recherche Scientifique
Role in Drug Synthesis
Precursor for Paclitaxel Production
XDT serves as an important intermediate in the semi-synthesis of paclitaxel. The conversion of 10-deacetylbaccatin III (10-DAB) into paclitaxel can be enhanced by utilizing XDT. Research has shown that enzymes such as 10-deacetylbaccatin III-10-O-acetyltransferase (DBAT) can catalyze the transformation of XDT into paclitaxel, significantly improving the yield and efficiency of this process. A study reported a mutant form of DBAT that increased catalytic efficiency by six times compared to the wild type, facilitating a one-pot conversion system for producing paclitaxel from XDT .
Separation Techniques
Efficient Separation Methods
The separation and enrichment of XDT and 10-DAB from plant extracts have been optimized using macroporous resins. A study demonstrated that AB-8 resin exhibited superior adsorption properties for both compounds, achieving substantial increases in their concentrations from initial low levels (0.053% and 0.2%) to 3.34% and 1.69%, respectively. This method not only enhances recovery rates but also streamlines the purification process necessary for further applications in drug synthesis .
Resin Type | Adsorption Capacity | Initial Concentration | Final Concentration | Recovery Rate |
---|---|---|---|---|
AB-8 | High | 0.053% (XDT) | 3.34% | 85.85% |
0.2% (10-DAB) | 1.69% | 52.78% |
Biochemical Studies
Enzymatic Conversion Studies
Research into the enzymatic conversion of XDT has revealed its potential for biotechnological applications. The use of glycoside hydrolases to convert XDT into more active forms like 10-deacetyltaxol has been explored, providing insights into the biochemical pathways involved in taxoid biosynthesis . These studies are crucial for developing environmentally friendly methods for producing high-value pharmaceuticals from natural products.
Mécanisme D'action
The mechanism of action of 7-Xylosyl-10-deacetylbaccatin III involves its conversion into 10-deacetyltaxol, which is then used in the semi-synthesis of paclitaxel. Paclitaxel exerts its effects by stabilizing microtubules and preventing their depolymerization, leading to mitotic arrest and apoptosis in cancer cells .
Comparaison Avec Des Composés Similaires
Comparison with Structurally Related Compounds
Structural and Functional Differences
The table below summarizes key structural and functional distinctions between 7-Xylosyl-10-deacetylbaccatin III and related taxanes:
Pharmacological and Industrial Relevance
- Bioactivity : Unlike paclitaxel, this compound lacks direct cytotoxicity but is pivotal as a biosynthetic precursor .
- Purification Efficiency : Chromatographic methods achieve 62.99% purity for this compound from crude Taxus extracts, outperforming 10-DAB III (83.24%) and paclitaxel (91.52%) .
- Stability : The xylosyl group confers stability under acidic conditions, facilitating storage and transport compared to labile taxanes like 10-DAB III .
Key Research Findings
Microbial Processing : Moraxella sp. strains hydrolyze xylosyltaxanes (e.g., this compound) with >90% efficiency, enabling scalable 10-DAB III production .
Synthetic Applications : Semisynthesis routes using this compound reduce reliance on natural paclitaxel extraction, addressing supply shortages .
Structural Analogues : Derivatives like 7-epi-19-Hydroxy-10-deacetylbaccatin III (C₂₉H₃₆O₁₁) exhibit unique cytotoxic profiles but require further pharmacological validation .
Activité Biologique
7-Xylosyl-10-deacetylbaccatin III is a significant compound derived from the Taxus species, particularly Taxus brevifolia. It plays a crucial role in the biosynthesis of paclitaxel, a widely used anticancer drug. This article explores the biological activity of this compound, including its mechanisms of action, biochemical properties, and relevant research findings.
Target and Mode of Action
The primary target of this compound is the microtubule structures within cells. Similar to paclitaxel, this compound binds to the β-subunit of tubulin, stabilizing microtubules and preventing their depolymerization. This interaction disrupts the normal function of microtubules, leading to cell cycle arrest in the G2/M phase and ultimately inducing apoptosis in cancer cells.
Biochemical Pathways
The compound undergoes bioconversion into 10-deacetyltaxol, which is a key precursor for the semi-synthesis of paclitaxel. The bioconversion process has been optimized in laboratory settings to enhance yield and efficiency.
Cellular Effects
The bioconversion product, paclitaxel, is known for its ability to induce mitotic arrest by stabilizing microtubules. This characteristic makes this compound valuable in cancer treatment research .
Chemical Reactions
This compound can undergo various chemical reactions including oxidation, reduction, and substitution. These reactions are essential for generating derivatives like 10-deacetyltaxol. Common reagents used include dimethyl sulfoxide and specific enzymes such as glycoside hydrolase.
Case Studies
-
Bacterial Bioconversion:
A study highlighted a Moraxella sp. strain capable of utilizing this compound through enzymatic activity that removes the xylosyl group. This process enhances the availability of 10-deacetylbaccatin III for further synthesis into paclitaxel . -
Transcriptome Analysis:
Research involving Taxus yunnanensis indicated that specific transcription factors are involved in paclitaxel biosynthesis, influencing the accumulation of this compound across different plant provenances . -
Enzymatic Activity:
Nocardioides luteus was identified as having enzyme activity that converts this compound into other useful derivatives with significant yields, demonstrating potential for industrial applications in drug synthesis .
Comparative Analysis
Compound | Role in Synthesis | Biological Activity |
---|---|---|
This compound | Precursor for paclitaxel | Induces apoptosis via microtubule stabilization |
Paclitaxel | Anticancer drug | Stabilizes microtubules to prevent cell division |
10-Deacetylbaccatin III | Intermediate in synthesis | Similar mechanism to paclitaxel |
Q & A
Basic Research Questions
Q. What analytical methods are recommended for identifying and quantifying 7-Xylosyl-10-deacetylbaccatin III in Taxus extracts?
- Methodological Answer : High-performance liquid chromatography (HPLC) with reversed-phase columns (e.g., EGHRA@SiO₂) is standard. Mobile phases like acetonitrile-water (47:53 v/v) at 15 mL/min flow rate achieve baseline separation. Validation includes peak purity assessment via diode-array detection (DAD) and comparison with reference standards (≥98% purity) . Mass spectrometry (MS) and nuclear magnetic resonance (NMR) further confirm structural identity .
Q. How does this compound contribute to paclitaxel biosynthesis?
- Methodological Answer : It acts as a precursor in the enzymatic pathway catalyzed by xylosyltransferases (XDT) and deacetylases. Key steps include xylose moiety addition at the C7 position and subsequent deacetylation. Researchers use heterologous expression systems (e.g., E. coli or yeast) to validate enzyme activity via kinetic assays and substrate-specificity tests .
Q. What spectroscopic techniques are critical for structural elucidation?
- Methodological Answer :
- NMR : Assigns proton/carbon shifts, particularly for xylosyl and acetyl groups.
- MS : Confirms molecular weight (C₃₄H₄₄O₁₄, MW 676.70) and fragmentation patterns .
- IR : Identifies functional groups (e.g., hydroxyl, carbonyl).
Reference standards must comply with pharmacopeial guidelines (e.g., USP, EP) for traceability .
Advanced Research Questions
Q. How can chromatographic conditions be optimized to resolve this compound from co-eluting taxanes?
- Methodological Answer :
- Column Selection : EGHRA@SiO₂ columns exhibit superior spatial selectivity due to phenanthrene-like bonding monomers, outperforming C18 columns for polar taxanes .
- Mobile Phase : Gradient elution with acetonitrile-water (adjusting ratios from 45:55 to 50:50) minimizes peak overlap.
- Validation : Spike recovery tests and inter-laboratory reproducibility studies ensure robustness.
Q. What experimental approaches resolve contradictions in reported enzymatic activities of xylosyltransferases (XDT)?
- Methodological Answer :
- Comparative Kinetics : Measure and across homologs (e.g., Taxus chinensis vs. T. brevifolia).
- Isotopic Labeling : Use -labeled substrates to track regioselectivity in xylose transfer.
- Genetic Knockouts : CRISPR/Cas9-edited plant lines validate in vivo relevance of XDT isoforms .
Q. What strategies enhance the stability of this compound during storage?
- Methodological Answer :
- Lyophilization : Reduces hydrolytic degradation.
- Inert Atmosphere : Store under argon at -20°C to prevent oxidation.
- Purity Monitoring : Regular HPLC checks (≥98% purity threshold) prevent batch variability .
Q. How do structural modifications at C7/C10 positions affect bioactivity?
- Methodological Answer :
- Synthetic Analogs : Introduce fluoro/trichloroethoxy groups via benzoylation (e.g., 2-(3-fluoro-benzoyl) derivatives) .
- Crystallography : X-ray diffraction reveals conformational changes impacting microtubule-binding affinity.
- In Silico Docking : Molecular dynamics simulations predict interactions with β-tubulin.
Q. Data Contradiction Analysis
Q. Why do purification yields of this compound vary across studies?
- Methodological Answer :
- Source Material : Variability in Taxus species (e.g., T. baccata vs. T. cuspidata) affects precursor abundance.
- Extraction Protocols : Ethanol vs. methanol extraction impacts solubility; sequential liquid-liquid partitioning improves recovery .
- Column Efficiency : EGHRA@SiO₂ achieves 62.99% purity improvement in one step, but older methods (e.g., silica gel) underperform .
Propriétés
IUPAC Name |
[(1S,2S,3R,4S,7R,9S,10S,12R,15S)-4-acetyloxy-1,12,15-trihydroxy-10,14,17,17-tetramethyl-11-oxo-9-[(2S,3R,4S,5R)-3,4,5-trihydroxyoxan-2-yl]oxy-6-oxatetracyclo[11.3.1.03,10.04,7]heptadec-13-en-2-yl] benzoate | |
---|---|---|
Source | PubChem | |
URL | https://pubchem.ncbi.nlm.nih.gov | |
Description | Data deposited in or computed by PubChem | |
InChI |
InChI=1S/C34H44O14/c1-15-18(36)12-34(43)28(47-29(42)17-9-7-6-8-10-17)26-32(5,27(41)24(39)22(15)31(34,3)4)20(11-21-33(26,14-45-21)48-16(2)35)46-30-25(40)23(38)19(37)13-44-30/h6-10,18-21,23-26,28,30,36-40,43H,11-14H2,1-5H3/t18-,19+,20-,21+,23-,24+,25+,26-,28-,30-,32+,33-,34+/m0/s1 | |
Source | PubChem | |
URL | https://pubchem.ncbi.nlm.nih.gov | |
Description | Data deposited in or computed by PubChem | |
InChI Key |
PAYIYFPUFSJTDA-HEJNHQNCSA-N | |
Source | PubChem | |
URL | https://pubchem.ncbi.nlm.nih.gov | |
Description | Data deposited in or computed by PubChem | |
Canonical SMILES |
CC1=C2C(C(=O)C3(C(CC4C(C3C(C(C2(C)C)(CC1O)O)OC(=O)C5=CC=CC=C5)(CO4)OC(=O)C)OC6C(C(C(CO6)O)O)O)C)O | |
Source | PubChem | |
URL | https://pubchem.ncbi.nlm.nih.gov | |
Description | Data deposited in or computed by PubChem | |
Isomeric SMILES |
CC1=C2[C@H](C(=O)[C@@]3([C@H](C[C@@H]4[C@]([C@H]3[C@@H]([C@@](C2(C)C)(C[C@@H]1O)O)OC(=O)C5=CC=CC=C5)(CO4)OC(=O)C)O[C@H]6[C@@H]([C@H]([C@@H](CO6)O)O)O)C)O | |
Source | PubChem | |
URL | https://pubchem.ncbi.nlm.nih.gov | |
Description | Data deposited in or computed by PubChem | |
Molecular Formula |
C34H44O14 | |
Source | PubChem | |
URL | https://pubchem.ncbi.nlm.nih.gov | |
Description | Data deposited in or computed by PubChem | |
Molecular Weight |
676.7 g/mol | |
Source | PubChem | |
URL | https://pubchem.ncbi.nlm.nih.gov | |
Description | Data deposited in or computed by PubChem | |
Retrosynthesis Analysis
AI-Powered Synthesis Planning: Our tool employs the Template_relevance Pistachio, Template_relevance Bkms_metabolic, Template_relevance Pistachio_ringbreaker, Template_relevance Reaxys, Template_relevance Reaxys_biocatalysis model, leveraging a vast database of chemical reactions to predict feasible synthetic routes.
One-Step Synthesis Focus: Specifically designed for one-step synthesis, it provides concise and direct routes for your target compounds, streamlining the synthesis process.
Accurate Predictions: Utilizing the extensive PISTACHIO, BKMS_METABOLIC, PISTACHIO_RINGBREAKER, REAXYS, REAXYS_BIOCATALYSIS database, our tool offers high-accuracy predictions, reflecting the latest in chemical research and data.
Strategy Settings
Precursor scoring | Relevance Heuristic |
---|---|
Min. plausibility | 0.01 |
Model | Template_relevance |
Template Set | Pistachio/Bkms_metabolic/Pistachio_ringbreaker/Reaxys/Reaxys_biocatalysis |
Top-N result to add to graph | 6 |
Feasible Synthetic Routes
Avertissement et informations sur les produits de recherche in vitro
Veuillez noter que tous les articles et informations sur les produits présentés sur BenchChem sont destinés uniquement à des fins informatives. Les produits disponibles à l'achat sur BenchChem sont spécifiquement conçus pour des études in vitro, qui sont réalisées en dehors des organismes vivants. Les études in vitro, dérivées du terme latin "in verre", impliquent des expériences réalisées dans des environnements de laboratoire contrôlés à l'aide de cellules ou de tissus. Il est important de noter que ces produits ne sont pas classés comme médicaments et n'ont pas reçu l'approbation de la FDA pour la prévention, le traitement ou la guérison de toute condition médicale, affection ou maladie. Nous devons souligner que toute forme d'introduction corporelle de ces produits chez les humains ou les animaux est strictement interdite par la loi. Il est essentiel de respecter ces directives pour assurer la conformité aux normes légales et éthiques en matière de recherche et d'expérimentation.