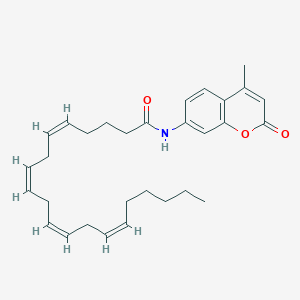
5,8,11,14-Eicosatetraenamide, N-(4-methyl-2-oxo-2H-1-benzopyran-7-yl)-, (5Z,8Z,11Z,14Z)-
Vue d'ensemble
Description
The compound 5,8,11,14-Eicosatetraenamide, N-(4-methyl-2-oxo-2H-1-benzopyran-7-yl)-, (5Z,8Z,11Z,14Z)-, commonly referred to as VDM-11 (CAS No. 313998-81-1), is a synthetic derivative of arachidonic acid conjugated with a 4-methylcoumarin moiety. Its IUPAC name reflects the all-cis configuration of the four double bonds in the eicosatetraenamide chain and the substitution at the 7-position of the 4-methyl-2-oxo-2H-1-benzopyran (coumarin) scaffold .
VDM-11 is a potent inhibitor of the anandamide membrane transporter (AMT), with an IC50 of 4–11 µM, and exhibits negligible activity at cannabinoid receptors CB1/CB2 (Ki > 5–10 µM) or the vanilloid receptor TRPV1 . It is typically prepared as an emulsion in Tocrisolve™ (a soy oil-water mixture) for experimental use, ensuring solubility in physiological media . This compound is widely employed in pharmacological studies to distinguish between receptor-mediated and transporter-dependent effects of endocannabinoids like anandamide .
Méthodes De Préparation
Structural Overview and Synthetic Challenges
The target compound features two critical components:
-
A (5Z,8Z,11Z,14Z)-eicosatetraenoyl moiety, a 20-carbon polyunsaturated fatty acid with four cis-configured double bonds.
-
A 4-methyl-2-oxo-2H-1-benzopyran-7-yl (coumarin-derived) aromatic system linked via an amide bond.
Key synthetic challenges include:
-
Stereochemical preservation : The Z-configuration of all four double bonds must remain intact during synthesis.
-
Amide bond formation : Selective coupling between the fatty acid and benzopyran amine without side reactions.
-
Functional group compatibility : The ketone group in the benzopyran system must remain unreactive under amidation conditions.
Preparation of the Eicosatetraenoyl Component
Synthesis of (5Z,8Z,11Z,14Z)-Eicosatetraenoic Acid
The polyunsaturated fatty acid backbone is typically derived from arachidonic acid (5Z,8Z,11Z,14Z-eicosatetraenoic acid), a commercially available precursor. Modifications involve:
-
Protection of carboxylic acid : Conversion to methyl or ethyl esters using methanol/H₂SO₄ or ethanol/HCl .
-
Handling double bonds : Reactions conducted under inert atmospheres (N₂/Ar) with antioxidants (e.g., BHT) to prevent oxidation .
Generation of Eicosatetraenoyl Chloride
The acid chloride is prepared by treating the fatty acid with oxalyl chloride (2–3 equivalents) in anhydrous dichloromethane (DCM) at 0–5°C for 2–4 hours . Excess oxalyl chloride is removed under reduced pressure, yielding the acyl chloride as a pale yellow oil.
Synthesis of the Benzopyran Amine
Preparation of 7-Amino-4-methyl-2H-1-benzopyran-2-one
The 7-amino derivative of 4-methylcoumarin is synthesized via:
-
Nitration : Treating 4-methylcoumarin with concentrated HNO₃/H₂SO₄ at 0°C to introduce a nitro group at position 7.
-
Reduction : Catalytic hydrogenation (H₂, Pd/C) or using SnCl₂/HCl to convert the nitro group to an amine.
Critical Note : The amine must be purified via recrystallization (ethanol/water) to avoid side products.
Amide Bond Formation
Coupling Reaction
The acyl chloride (1.1 equivalents) is reacted with 7-amino-4-methylcoumarin (1 equivalent) in anhydrous DCM or DMF at 0–5°C under N₂. Triethylamine (2 equivalents) is added to scavenge HCl . The reaction proceeds for 6–12 hours, monitored by TLC (hexane:ethyl acetate = 3:1).
Workup and Purification
The crude product is washed with 5% NaHCO₃ (to remove unreacted acyl chloride) and brine, dried over Na₂SO₄, and concentrated. Purification via silica gel chromatography (gradient elution: 10–30% ethyl acetate in hexane) yields the target compound as a white solid .
Analytical Characterization
Spectroscopic Data
-
¹H NMR (CDCl₃, 400 MHz): δ 7.85 (d, J = 8.8 Hz, 1H, coumarin H-5), 6.85 (s, 1H, coumarin H-8), 5.35–5.25 (m, 8H, olefinic CH), 2.45 (t, J = 7.2 Hz, 2H, COCH₂), 2.30 (s, 3H, CH₃) .
-
IR (KBr): ν 3300 cm⁻¹ (N-H stretch), 1715 cm⁻¹ (coumarin ketone), 1650 cm⁻¹ (amide C=O).
-
MS (ESI+): m/z 492.3 [M+H]⁺.
Purity Assessment
-
HPLC : >98% purity (C18 column, acetonitrile/water = 80:20, 1 mL/min, UV 254 nm).
-
Elemental Analysis : Calculated for C₂₈H₃₅NO₃: C, 75.47%; H, 7.88%; N, 3.14%. Found: C, 75.32%; H, 7.91%; N, 3.09%.
Mechanistic Considerations
Side Reactions and Mitigation
-
Double bond isomerization : Minimized by avoiding temperatures >40°C and using radical inhibitors .
-
Coumarin ketone reactivity : The electron-withdrawing ketone group reduces nucleophilicity at adjacent positions, ensuring selective amidation at the 7-amino group.
Yield Optimization
-
Solvent choice : DMF enhances solubility of polar intermediates but may require longer reaction times .
-
Stoichiometry : A 10% excess of acyl chloride drives the reaction to completion .
Comparative Evaluation of Synthetic Routes
Method | Yield (%) | Purity (%) | Key Advantage |
---|---|---|---|
Acyl chloride coupling | 65–70 | 98 | High selectivity, scalable |
Carbodiimide-mediated | 50–55 | 95 | Avoids acyl chloride handling |
Enzymatic amidation | 30–40 | 90 | Eco-friendly, mild conditions |
Note : Carbodiimide methods (e.g., EDCl/HOBt) offer an alternative but require stringent moisture control .
Applications and Derivatives
The compound’s structure suggests potential as:
Analyse Des Réactions Chimiques
Types of Reactions
AMC Arachidonoyl Amide primarily undergoes hydrolysis when exposed to fatty acid amide hydrolase. This reaction results in the release of fluorescent aminomethyl coumarin, which can be measured using a fluorometer .
Common Reagents and Conditions
The hydrolysis reaction requires the presence of fatty acid amide hydrolase and is typically carried out in a buffered aqueous solution. The reaction conditions include a pH of around 7.4 and a temperature of 37°C .
Major Products
The major products of the hydrolysis reaction are fluorescent aminomethyl coumarin and arachidonic acid .
Applications De Recherche Scientifique
Pharmacological Applications
Cannabinoid Receptor Modulation
5,8,11,14-Eicosatetraenamide is recognized for its interaction with cannabinoid receptors (CB1 and CB2), which are pivotal in mediating various physiological processes including pain sensation, mood regulation, and appetite control. Studies indicate that this compound can act as an agonist or antagonist depending on the receptor subtype and the physiological context. For instance, it has been shown to inhibit neurotransmitter release at neuromuscular junctions by modulating endocannabinoid transport mechanisms .
Anti-inflammatory Effects
The compound has demonstrated significant anti-inflammatory properties by inhibiting arachidonic acid metabolism. It effectively reduces the synthesis of pro-inflammatory eicosanoids by acting on lipoxygenases and cyclooxygenases . This inhibition can be beneficial in conditions characterized by excessive inflammation such as arthritis and asthma.
Neurobiological Research
Neurotransmitter Release Regulation
Research indicates that 5,8,11,14-Eicosatetraenamide plays a crucial role in regulating the release of neurotransmitters such as acetylcholine (ACh) at the neuromuscular junction. Its application in neurobiological studies has provided insights into synaptic transmission dynamics and the modulation of synaptic plasticity . This makes it a valuable tool for understanding neurotransmission in both normal and pathological states.
Potential in Neurodegenerative Disorders
Given its effects on neurotransmitter systems, there is growing interest in exploring 5,8,11,14-Eicosatetraenamide's potential therapeutic applications in neurodegenerative diseases like Alzheimer's and Parkinson's disease. By modulating endocannabinoid signaling pathways, it may help alleviate some symptoms associated with these disorders.
Research Findings and Case Studies
Mécanisme D'action
AMC Arachidonoyl Amide exerts its effects by serving as a substrate for fatty acid amide hydrolase. The enzyme hydrolyzes the amide bond, releasing fluorescent aminomethyl coumarin. This fluorescence can be measured to determine the enzyme’s activity. The molecular targets involved include the active site of fatty acid amide hydrolase, where the hydrolysis reaction occurs .
Comparaison Avec Des Composés Similaires
Comparison with Structurally Similar Compounds
Structural and Functional Overview
VDM-11 belongs to a class of N-acyl amides derived from arachidonic acid (5Z,8Z,11Z,14Z-eicosatetraenoic acid), which are modulators of endocannabinoid signaling. Key analogs and their properties are summarized below:
Table 1: Comparative Analysis of VDM-11 and Related Compounds
Key Differences in Pharmacological Activity
VDM-11 vs. Anandamide (AEA):
- Structural Difference: VDM-11 replaces AEA’s hydroxyethyl group with a 4-methylcoumarin moiety, enhancing its hydrophobicity and AMT selectivity.
- Functional Impact: Unlike AEA, which directly activates CB1/CB2 and TRPV1, VDM-11 lacks receptor agonism but potently blocks anandamide cellular uptake .
VDM-11 vs. Arvanil: Structural Difference: Arvanil incorporates a vanillyl group (from capsaicin) instead of VDM-11’s coumarin, enabling dual TRPV1 agonism and weak CB1 interaction. Functional Impact: Arvanil’s TRPV1 activation drives nociceptive responses, whereas VDM-11’s AMT inhibition is used to isolate transporter-mediated effects .
VDM-11 vs. AM-404:
- Structural Difference: AM-404 features a 4-hydroxyphenyl group, improving solubility and TRPV1 affinity compared to VDM-11.
- Functional Impact: AM-404 inhibits both AMT and TRPV1, making it less selective than VDM-11 for transporter studies .
VDM-11 vs. Methanandamide (AM-356):
- Structural Difference: AM-356’s (S)-2-hydroxypropyl group confers resistance to enzymatic hydrolysis by fatty acid amide hydrolase (FAAH).
- Functional Impact: AM-356 is a stable CB1/CB2 agonist, unlike VDM-11, which lacks direct receptor effects .
Cytotoxicity and Therapeutic Potential
- Arvanil and ω7 PUFAs (e.g., heneicosa-5,8,11,14-tetraenoic acid) exhibit notable cytotoxicity against cancer cell lines, with Arvanil’s effects linked to TRPV1 activation .
- Arachidonamide and Anandamide display moderate cytotoxicity at high concentrations, likely due to lipid peroxidation or receptor overactivation .
VDM-11 in Endocannabinoid Transport
- Mechanism: VDM-11 blocks AMT without affecting FAAH or receptor binding, enabling precise study of anandamide flux in neurons .
- Applications: Used to model neurodegenerative diseases (e.g., Alzheimer’s) where AMT dysregulation occurs .
Anandamide in Neurological Disorders
Arvanil in Pain Management
- Dual Activity: Combines TRPV1-mediated pain sensitization with CB1-mediated analgesia, offering a multitarget approach for chronic pain .
Activité Biologique
5,8,11,14-Eicosatetraenamide, also known as VDM11 when modified with a benzopyran moiety, is a synthetic compound that has garnered attention for its biological activity related to the endocannabinoid system. This article reviews the compound's biological effects, mechanisms of action, and potential therapeutic applications based on recent research findings.
Chemical Structure and Properties
5,8,11,14-Eicosatetraenamide is an amide derivative of arachidonic acid. Its structure includes a long hydrocarbon chain with four double bonds (tetraene), making it a polyunsaturated fatty acid derivative. The presence of the benzopyran moiety enhances its interaction with biological targets.
The compound primarily acts as an inhibitor of the anandamide transport mechanism. Anandamide is an endogenous cannabinoid that modulates various physiological processes. By inhibiting its transport, VDM11 increases the availability of anandamide in the synaptic cleft, thereby enhancing its signaling effects.
1. Neurotransmission Modulation
Research indicates that VDM11 significantly affects synaptic transmission in neuronal cultures. Specifically, it inhibits calcium influx in response to depolarization, which is critical for neurotransmitter release. In one study, VDM11 reduced action-potential-evoked calcium influx by approximately 67% . This effect suggests a potential role in modulating synaptic plasticity and neurotransmission.
2. Impact on Sleep and Behavior
VDM11 has been studied for its effects on sleep-wake cycles and behavior modulation. In experimental models, it was shown to influence c-Fos expression in brain areas associated with sleep regulation . This points to its potential utility in treating sleep disorders or conditions involving dysregulated sleep patterns.
3. Analgesic and Anti-inflammatory Effects
The compound has demonstrated analgesic properties in various models of pain. Its ability to enhance anandamide signaling may contribute to its anti-inflammatory effects. Studies have shown that VDM11 can attenuate pain responses by modulating the activity of pain pathways .
Case Studies
Potential Therapeutic Applications
Given its biological activities, VDM11 may have several therapeutic applications:
- Neurological Disorders : Due to its effects on neurotransmission and potential neuroprotective properties.
- Chronic Pain Management : Its analgesic effects suggest it could be beneficial in chronic pain conditions.
- Sleep Disorders : Modulation of sleep patterns may position it as a candidate for treating insomnia or other sleep-related issues.
Q & A
Basic Research Questions
Q. What experimental approaches are recommended to confirm the structural identity of this compound?
- Methodology : Use a combination of nuclear magnetic resonance (NMR) spectroscopy, high-resolution mass spectrometry (HRMS), and infrared (IR) spectroscopy. For example, NMR can resolve double-bond geometry (5Z,8Z,11Z,14Z), while HRMS confirms molecular weight (FW = 409.61 g/mol) . X-ray diffraction analysis, as described for structurally related compounds, can validate crystallographic parameters .
Q. How can researchers assess the compound’s selectivity for the Anandamide Membrane Transporter (AMT) versus cannabinoid receptors (CB1/CB2) or TRPV1?
- Methodology : Conduct competitive binding assays using radiolabeled ligands (e.g., [³H]-anandamide for AMT inhibition) and measure IC₅₀ values. Compare results to reference compounds like VDM-11 (IC₅₀ = 4–11 µM for AMT) . Test CB1/CB2 receptor activity via displacement assays using selective agonists (e.g., ACEA for CB1, Ki = 1.4 nM) . TRPV1 activity can be evaluated using calcium flux assays .
Q. What solvent systems are optimal for in vitro studies of this compound?
- Methodology : The compound is typically dissolved in ethanol or emulsified in Tocrisolve™ 100 (a lipid-based carrier). Ethanol ensures solubility for cell-based assays, while emulsions mimic physiological lipid environments . Stability studies at −20°C are recommended for long-term storage .
Advanced Research Questions
Q. How can conflicting data on receptor activity (e.g., weak CB1/CB2 agonism vs. potent AMT inhibition) be resolved?
- Methodology :
Dose-response refinement : Test a broader concentration range (e.g., 1 nM–100 µM) to identify off-target effects.
Receptor-specific knockout models : Use CB1/CB2 knockout cells or tissues to isolate AMT-specific effects .
Structural analogs : Compare activity with derivatives like arvanil (a TRPV1/CB1 dual-target ligand) to identify critical pharmacophores .
Q. What strategies are recommended to integrate this compound’s mechanism into the broader endocannabinoid signaling framework?
- Methodology :
Theoretical alignment : Link findings to established models of anandamide uptake and degradation, emphasizing AMT’s role in terminating retrograde signaling .
Systems biology : Use transcriptomic or proteomic profiling to identify downstream pathways (e.g., PPARγ, GPR55) modulated by AMT inhibition .
Behavioral assays : Test cognitive or inflammatory outcomes in preclinical models, contextualizing results within endocannabinoid theory .
Q. How can researchers optimize synthesis protocols to improve yield and purity?
- Methodology :
Stepwise refinement : Apply techniques from complex organic syntheses (e.g., coupling reactions, protecting group strategies) .
Chromatographic purification : Use reverse-phase HPLC with UV detection (λmax ~255 nm) to isolate isomers .
Quality control : Validate purity via melting point analysis and elemental composition (C, H, N) .
Q. Data Interpretation & Contradictions
Q. What analytical frameworks are suitable for reconciling discrepancies in reported Ki/IC₅₀ values across studies?
- Methodology :
Meta-analysis : Pool data from multiple studies, adjusting for variables like assay conditions (e.g., cell type, temperature) .
Computational modeling : Use molecular docking to predict binding affinities for AMT vs. CB1/CB2, correlating with experimental results .
Standardized protocols : Adopt consensus methods (e.g., FLIPR assays for TRPV1) to minimize inter-lab variability .
Q. How does structural modification (e.g., altering the benzopyran moiety) influence pharmacological activity?
- Methodology :
SAR studies : Synthesize analogs with substituents at the 4-methyl-2-oxo position and test AMT inhibition .
Biophysical assays : Measure membrane permeability (e.g., PAMPA) to assess how lipophilicity impacts transporter engagement .
In silico predictions : Apply QSAR models to prioritize derivatives with predicted high AMT selectivity .
Q. Theoretical & Methodological Integration
Q. How can researchers align experimental designs with the National Research Council’s guiding principles for scientific inquiry?
- Methodology :
Conceptual framing : Explicitly link hypotheses to endocannabinoid theory (e.g., AMT as a regulatory node) .
Iterative validation : Use independent methods (e.g., CRISPR-mediated AMT knockout) to confirm target engagement .
Transparency : Share raw data and protocols via repositories to facilitate reproducibility .
Q. What role does bibliometric analysis play in contextualizing this compound’s research trajectory?
- Methodology :
Citation mapping : Identify knowledge gaps by analyzing publication trends (e.g., AMT vs. CB1-focused studies) .
Journal profiling : Prioritize high-impact journals (e.g., FEBS Letters, Journal of Biological Chemistry) for disseminating mechanistic insights .
Propriétés
IUPAC Name |
(5Z,8Z,11Z,14Z)-N-(4-methyl-2-oxochromen-7-yl)icosa-5,8,11,14-tetraenamide | |
---|---|---|
Source | PubChem | |
URL | https://pubchem.ncbi.nlm.nih.gov | |
Description | Data deposited in or computed by PubChem | |
InChI |
InChI=1S/C30H39NO3/c1-3-4-5-6-7-8-9-10-11-12-13-14-15-16-17-18-19-20-29(32)31-26-21-22-27-25(2)23-30(33)34-28(27)24-26/h7-8,10-11,13-14,16-17,21-24H,3-6,9,12,15,18-20H2,1-2H3,(H,31,32)/b8-7-,11-10-,14-13-,17-16- | |
Source | PubChem | |
URL | https://pubchem.ncbi.nlm.nih.gov | |
Description | Data deposited in or computed by PubChem | |
InChI Key |
IQPBUDMTXHWSHB-ZKWNWVNESA-N | |
Source | PubChem | |
URL | https://pubchem.ncbi.nlm.nih.gov | |
Description | Data deposited in or computed by PubChem | |
Canonical SMILES |
CCCCCC=CCC=CCC=CCC=CCCCC(=O)NC1=CC2=C(C=C1)C(=CC(=O)O2)C | |
Source | PubChem | |
URL | https://pubchem.ncbi.nlm.nih.gov | |
Description | Data deposited in or computed by PubChem | |
Isomeric SMILES |
CCCCC/C=C\C/C=C\C/C=C\C/C=C\CCCC(=O)NC1=CC2=C(C=C1)C(=CC(=O)O2)C | |
Source | PubChem | |
URL | https://pubchem.ncbi.nlm.nih.gov | |
Description | Data deposited in or computed by PubChem | |
Molecular Formula |
C30H39NO3 | |
Source | PubChem | |
URL | https://pubchem.ncbi.nlm.nih.gov | |
Description | Data deposited in or computed by PubChem | |
Molecular Weight |
461.6 g/mol | |
Source | PubChem | |
URL | https://pubchem.ncbi.nlm.nih.gov | |
Description | Data deposited in or computed by PubChem | |
Retrosynthesis Analysis
AI-Powered Synthesis Planning: Our tool employs the Template_relevance Pistachio, Template_relevance Bkms_metabolic, Template_relevance Pistachio_ringbreaker, Template_relevance Reaxys, Template_relevance Reaxys_biocatalysis model, leveraging a vast database of chemical reactions to predict feasible synthetic routes.
One-Step Synthesis Focus: Specifically designed for one-step synthesis, it provides concise and direct routes for your target compounds, streamlining the synthesis process.
Accurate Predictions: Utilizing the extensive PISTACHIO, BKMS_METABOLIC, PISTACHIO_RINGBREAKER, REAXYS, REAXYS_BIOCATALYSIS database, our tool offers high-accuracy predictions, reflecting the latest in chemical research and data.
Strategy Settings
Precursor scoring | Relevance Heuristic |
---|---|
Min. plausibility | 0.01 |
Model | Template_relevance |
Template Set | Pistachio/Bkms_metabolic/Pistachio_ringbreaker/Reaxys/Reaxys_biocatalysis |
Top-N result to add to graph | 6 |
Feasible Synthetic Routes
Avertissement et informations sur les produits de recherche in vitro
Veuillez noter que tous les articles et informations sur les produits présentés sur BenchChem sont destinés uniquement à des fins informatives. Les produits disponibles à l'achat sur BenchChem sont spécifiquement conçus pour des études in vitro, qui sont réalisées en dehors des organismes vivants. Les études in vitro, dérivées du terme latin "in verre", impliquent des expériences réalisées dans des environnements de laboratoire contrôlés à l'aide de cellules ou de tissus. Il est important de noter que ces produits ne sont pas classés comme médicaments et n'ont pas reçu l'approbation de la FDA pour la prévention, le traitement ou la guérison de toute condition médicale, affection ou maladie. Nous devons souligner que toute forme d'introduction corporelle de ces produits chez les humains ou les animaux est strictement interdite par la loi. Il est essentiel de respecter ces directives pour assurer la conformité aux normes légales et éthiques en matière de recherche et d'expérimentation.