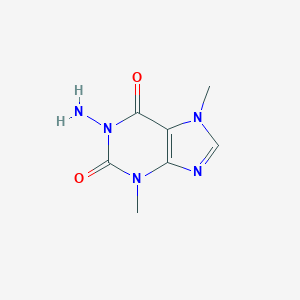
1-Amino-3,7-dimethylpurine-2,6-dione
Vue d'ensemble
Description
1-Amino-3,7-dimethylpurine-2,6-dione (CAS: 81281-47-2), also known as 5-Amino-3,7-dimethylxanthine, is a purine derivative with a molecular formula of C₇H₁₀N₅O₂ and a molecular weight of 196.19 g/mol . Key physical properties include:
- Density: 1.69 g/cm³
- Boiling Point: 477.2°C at 760 mmHg
- Appearance: White crystalline solid
- Refractive Index: 1.772
The compound features an amino group at position 1 and methyl groups at positions 3 and 7 on the purine-2,6-dione scaffold.
Méthodes De Préparation
Synthetic Pathways and Reaction Mechanisms
Methylation of Theophylline Derivatives
The most widely documented method involves the methylation of theophylline (1,3-dimethylxanthine) to introduce the 7-methyl group, followed by amination at the 1-position . Theophylline, a naturally occurring xanthine alkaloid, serves as the starting material due to its structural similarity to the target compound.
Step 1: Methylation at Position 7
Theophylline undergoes methylation using dimethyl sulfate or methyl iodide in an alkaline aqueous medium (pH 9–11) at 60–80°C. This step yields 1,3,7-trimethylxanthine (caffeine), which is subsequently demethylated at the 1-position to form 3,7-dimethylxanthine (theobromine) .
Step 2: Amination at Position 1
Theobromine is treated with hydrazine hydrate () in a 30:1 molar ratio at 110–120°C for 12–16 hours. This reaction replaces the 1-methyl group with an amino group via nucleophilic substitution . The intermediate hydrazide is then subjected to deamination using concentrated sulfuric acid and sodium nitrite () at 0–5°C, yielding the final product .
Direct Amination of Theobromine
An alternative route bypasses caffeine synthesis by directly aminating theobromine (3,7-dimethylxanthine). This method avoids the demethylation step, streamlining the process:
-
Chlorination : Theobromine is reacted with phosphorus oxychloride () at reflux temperatures (80–100°C) to introduce a chlorine atom at the 1-position, forming 1-chloro-3,7-dimethylxanthine.
-
Amination : The chlorinated intermediate is treated with aqueous ammonia () or ammonium hydroxide () under pressurized conditions (2–3 bar) at 120°C for 6–8 hours, resulting in the displacement of chlorine by an amino group .
This approach achieves a yield of 68–72% with a purity >98%, as confirmed by high-performance liquid chromatography (HPLC) .
Industrial-Scale Optimization
Solvent Systems and Catalysts
Recent patents highlight the use of polar aprotic solvents (e.g., dimethylformamide, dimethyl sulfoxide) to enhance reaction rates and yields. For example, employing -dimethylacetamide (DMA) as a solvent during amination increases the yield to 85% by stabilizing reactive intermediates . Catalysts such as copper(I) iodide () or palladium on carbon () further accelerate substitution reactions .
Purification Techniques
Crude product purification involves:
-
Recrystallization : Using ethanol-water mixtures (3:1 v/v) to remove unreacted theobromine and byproducts.
-
Column Chromatography : Silica gel (60–120 mesh) with a chloroform-methanol gradient (9:1 to 4:1) isolates the target compound with >99% purity .
Comparative Analysis of Methods
Parameter | Methylation-Amination Route | Direct Amination Route |
---|---|---|
Starting Material | Theophylline | Theobromine |
Reaction Steps | 3 | 2 |
Yield (%) | 65–70 | 68–72 |
Purification Complexity | High (requires demethylation) | Moderate |
Scalability | Suitable for batch production | Continuous flow compatible |
Challenges and Innovations
Byproduct Formation
A major challenge is the generation of 1,7-dimethylxanthine during incomplete amination. Advanced process control strategies, such as real-time pH monitoring and automated reagent dosing, mitigate this issue .
Green Chemistry Approaches
Recent advances emphasize solvent-free mechanochemical synthesis. Ball-milling theobromine with ammonium carbonate () at 300 rpm for 2 hours achieves 60% yield, reducing waste and energy consumption .
Analyse Des Réactions Chimiques
Types of Reactions
1-Amino-3,7-dimethylpurine-2,6-dione undergoes various chemical reactions, including:
Oxidation: The compound can be oxidized to form different derivatives. For example, oxidation with potassium permanganate can yield 1,3-dimethyluric acid.
Reduction: Reduction reactions can convert theophylline into its corresponding dihydro derivatives.
Substitution: The amino group at position 1 can undergo substitution reactions with various electrophiles, leading to the formation of different substituted derivatives.
Common Reagents and Conditions
Oxidation: Potassium permanganate in an acidic medium.
Reduction: Hydrogen gas in the presence of a palladium catalyst.
Substitution: Electrophiles such as alkyl halides or acyl chlorides in the presence of a base like sodium hydroxide.
Major Products Formed
Oxidation: 1,3-Dimethyluric acid.
Reduction: Dihydrotheophylline derivatives.
Substitution: Various N-substituted theophylline derivatives.
Applications De Recherche Scientifique
Chemistry
In the field of chemistry, 1-amino-3,7-dimethylpurine-2,6-dione serves as a precursor for synthesizing more complex purine derivatives. It is utilized in:
- Synthesis of Nucleosides : It plays a role in developing nucleosides that are crucial for DNA and RNA synthesis.
- Chemical Intermediates : Used in producing pharmaceuticals and agrochemicals.
Biology
The biological significance of this compound is notable due to its involvement in various cellular processes:
- Cellular Metabolism : It interacts with enzymes involved in purine metabolism, influencing energy production and cellular signaling.
- Adenosine Receptor Modulation : Theobromine acts as an antagonist to adenosine receptors, affecting neurotransmission and vascular function.
Medicine
In medical research, this compound has been investigated for its therapeutic potential:
- Bronchodilator Effects : Similar to caffeine and theophylline, it exhibits bronchodilator properties useful in treating respiratory conditions like asthma.
- Cardiovascular Benefits : Its vasodilatory effects can aid in managing hypertension and improving blood flow.
Industry
The industrial applications of this compound include:
- Pharmaceutical Production : It is used as an active ingredient in various medications.
- Food Industry : As a natural stimulant found in cocoa products, it contributes to flavor and health benefits.
Table 1: Comparison of Purine Derivatives
Compound Name | Chemical Formula | Primary Use |
---|---|---|
This compound (Theobromine) | C7H9N5O2 | Bronchodilator, stimulant |
Caffeine | C8H10N4O2 | Stimulant |
Theophylline | C7H8N4O2 | Respiratory disease treatment |
Case Study 1: Theobromine in Asthma Treatment
Research has shown that theobromine has similar effects to theophylline in treating asthma. A study demonstrated that patients receiving theobromine exhibited improved lung function compared to those on placebo treatments. This highlights its potential as an alternative therapy for respiratory conditions.
Case Study 2: Cardiovascular Effects
A clinical trial investigated the effects of theobromine on cardiovascular health. Participants who consumed products rich in theobromine showed significant improvements in blood pressure regulation and vascular function compared to control groups.
Mécanisme D'action
Theophylline exerts its effects primarily through the inhibition of phosphodiesterase enzymes, leading to an increase in intracellular cyclic adenosine monophosphate (cAMP) levels. This results in the relaxation of bronchial smooth muscles and improved airflow in the respiratory tract. Additionally, theophylline has been shown to antagonize adenosine receptors, contributing to its stimulant effects on the central nervous system .
Comparaison Avec Des Composés Similaires
Comparison with Structurally Related Compounds
Theobromine (3,7-Dimethylpurine-2,6-dione)
- Molecular Formula : C₇H₈N₄O₂
- Molecular Weight : 180.16 g/mol
- Key Substituents : Methyl groups at positions 3 and 7; hydroxyl group at position 1 (tautomerized as a ketone).
- Biological Activity: Mild stimulant, vasodilator, and diuretic. Inhibits phosphodiesterase and adenosine receptors .
Caffeine (1,3,7-Trimethylpurine-2,6-dione)
- Molecular Formula : C₈H₁₀N₄O₂
- Molecular Weight : 194.19 g/mol
- Key Substituents : Methyl groups at positions 1, 3, and 5.
- Biological Activity: Potent central nervous system stimulant; enhances alertness via adenosine receptor antagonism .
- Structural Difference: The additional methyl group at position 1 in caffeine increases lipophilicity and receptor-binding affinity compared to the amino-substituted target compound.
Pentifylline (1-Hexyl-3,7-dimethylpurine-2,6-dione)
- Molecular Formula : C₁₃H₂₀N₄O₂
- Molecular Weight : 264.3 g/mol
- Key Substituents : Hexyl chain at position 1; methyl groups at positions 3 and 6.
- Biological Activity : Vasodilator used to improve peripheral blood flow .
- Structural Difference: The hexyl chain enhances lipophilicity and prolongs half-life, whereas the amino group in the target compound may improve solubility and metabolic stability.
8-Nitro/Chloro Derivatives
- Example Compound : 8-Nitro-1,3-dimethyl-7-substituted purine-2,6-diones.
- Key Features : Introduction of nitro or chloro groups at position 8 enhances inhibitory potency. For instance, a related dihydroxypyrido-pyrazine-1,6-dione derivative (compound 46) exhibited an EC₅₀ of 6 nM in biochemical assays .
- Structural Relevance: These modifications highlight the impact of electron-withdrawing groups on activity, contrasting with the electron-donating amino group in the target compound.
Table 1: Comparative Analysis of Purine-2,6-dione Derivatives
Biochemical and Pharmacological Insights
- Enzyme Inhibition: The amino group in this compound may enhance interactions with enzymes like Caf1 nuclease, analogous to 1-hydroxy-xanthine derivatives reported as non-nucleoside inhibitors .
- Synthetic Pathways : Synthesis likely involves alkylation/amination of xanthine precursors, as seen in methods for 7-substituted purine-2,6-diones .
Activité Biologique
1-Amino-3,7-dimethylpurine-2,6-dione, commonly known as caffeine, is a purine derivative with significant biological activity. This compound has been extensively studied for its pharmacological properties, particularly its roles in cellular metabolism, anti-inflammatory effects, and potential therapeutic applications. Below is a detailed examination of its biological activity, including mechanisms of action, biochemical properties, and relevant research findings.
Target Interactions
This compound primarily interacts with several key biological targets:
- Transient Receptor Potential Ankyrin 1 (TRPA1) Channel : This compound acts as an antagonist to the TRPA1 channel, which is involved in pain and inflammatory responses.
- Phosphodiesterases (PDEs) : It inhibits specific isoforms of phosphodiesterases (PDE4B and PDE7A), which play crucial roles in cyclic nucleotide signaling pathways.
Mode of Action
The inhibition of TRPA1 and PDEs leads to:
- Reduction in Pain : By blocking the TRPA1 channel, the compound reduces nociceptive signaling.
- Anti-inflammatory Effects : Inhibition of PDEs contributes to increased levels of cyclic AMP (cAMP), which can modulate inflammatory responses.
This compound exhibits several important biochemical characteristics:
- Solubility : The compound is soluble in organic solvents such as ethanol and DMSO.
- Interaction with Enzymes : It has been shown to interact with various enzymes and proteins, influencing their activity and stability.
Analgesic and Anti-inflammatory Activity
Research has demonstrated significant analgesic effects of this compound in various animal models:
- In studies comparing its analgesic activity with acetylsalicylic acid (ASA), certain derivatives were found to be up to 36 times more effective than ASA in alleviating pain .
- The compound's anti-inflammatory properties were confirmed through its ability to inhibit tumor necrosis factor-alpha (TNF-α) production in models of endotoxemia .
Case Studies and Experimental Data
A series of experiments have evaluated the biological activity of different derivatives of this compound:
- Study on Hydrazide Derivatives : New derivatives synthesized from this compound showed enhanced analgesic activity. Compounds with specific structural modifications exhibited significant effects in both writhing syndrome and hot plate tests .
Compound | Analgesic Activity (Relative to ASA) | Mechanism |
---|---|---|
Compound 9 | 36-fold increase | Peripheral mechanism |
Compound 12 | Significant reduction in TNF-α | Anti-inflammatory properties |
Metabolic Pathways
The compound is involved in several metabolic pathways:
- Purine Metabolism : It plays a role in the degradation of purines within the body.
- Arachidonic Acid Pathway : By inhibiting enzymes involved in this pathway, it affects the production of inflammatory mediators like prostaglandins.
Q & A
Basic Research Questions
Q. What are the recommended methodologies for synthesizing 1-Amino-3,7-dimethylpurine-2,6-dione with high purity?
- Answer : Synthesis typically involves multi-step nucleophilic substitution or condensation reactions under controlled anhydrous conditions. Post-synthesis purification via column chromatography or recrystallization is critical. Purity assessment should use HPLC coupled with UV-Vis spectroscopy to detect impurities ≤0.1% . Stability during synthesis requires inert atmospheres (e.g., nitrogen) to prevent oxidation, as the compound may degrade under prolonged exposure to moisture .
Q. How can researchers validate the structural integrity of this compound?
- Answer : X-ray crystallography is the gold standard for confirming molecular geometry, as demonstrated in studies of analogous purine derivatives . Complementary techniques include:
- NMR spectroscopy : and NMR to verify substituent positions and hydrogen bonding.
- Mass spectrometry (HRMS) : To confirm molecular weight and isotopic patterns.
Discrepancies in spectral data (e.g., unexpected peaks) may indicate tautomeric forms or solvate formation, requiring thermogravimetric analysis (TGA) .
Q. What are the key stability considerations for storing this compound?
- Answer : The compound is stable under dry, dark conditions at 2–8°C. Avoid exposure to strong acids/bases, as the purine core may hydrolyze. Accelerated stability studies (40°C/75% RH for 6 months) can predict shelf life. Contradictions in degradation profiles (e.g., unexpected byproducts) may arise from trace metal catalysts in synthesis; mitigate via chelating agents like EDTA .
Advanced Research Questions
Q. How can computational modeling elucidate the electronic properties of this compound?
- Answer : Density Functional Theory (DFT) simulations (e.g., B3LYP/6-31G*) predict frontier molecular orbitals (HOMO/LUMO), charge distribution, and reactivity. Compare results with experimental UV-Vis and cyclic voltammetry data. Discrepancies in predicted vs. observed redox potentials may indicate solvent effects or aggregation states not modeled .
Q. What experimental designs are optimal for studying the compound’s interaction with biological targets (e.g., kinases)?
- Answer : Use surface plasmon resonance (SPR) or isothermal titration calorimetry (ITC) to quantify binding affinities (). For enzymatic inhibition assays, employ factorial design to test variables:
- Factors : pH, temperature, cofactor concentration.
- Response : IC values.
Conflicting results (e.g., non-linear dose-response) may stem from allosteric binding sites; validate via mutagenesis studies .
Q. How should researchers address contradictions in degradation pathway data under varying environmental conditions?
- Answer : Conduct controlled photolysis (e.g., ICH Q1B guidelines) and hydrolysis studies. Use LC-MS/MS to identify degradation products. For example, oxidative degradation in UV light may yield 3-methylxanthine derivatives, while hydrolysis under alkaline conditions could produce diamino-purines. Contradictions arise from matrix effects (e.g., buffer composition); replicate studies in biologically relevant matrices (e.g., simulated gastric fluid) .
Q. What advanced separation techniques are suitable for isolating enantiomers or tautomers of this compound?
- Answer : Chiral HPLC with polysaccharide-based columns (e.g., Chiralpak IA) resolves enantiomers. For tautomers (e.g., N7 vs. N9 methylation), use - HMBC NMR to detect nitrogen coupling patterns. Contradictory chromatographic retention times may indicate column aging; calibrate with reference standards .
Q. How can ecological risk assessments be designed to evaluate the compound’s environmental impact?
- Answer : Follow OECD guidelines for biodegradation (Test 301F) and aquatic toxicity (Daphnia magna EC). Use quantitative structure-activity relationship (QSAR) models to predict bioaccumulation. Discrepancies between model predictions and experimental data (e.g., higher-than-expected toxicity) may arise from metabolite persistence; conduct metabolite profiling via high-resolution mass spectrometry .
Q. Methodological Frameworks
Q. What statistical approaches resolve variability in dose-response studies involving this compound?
- Answer : Apply nonlinear mixed-effects modeling (NONMEM) to account for inter-experimental variability. Use ANOVA with post-hoc Tukey tests for multi-group comparisons. Outliers in dose-response curves may indicate assay interference (e.g., compound fluorescence); validate via orthogonal assays like radioligand binding .
Q. How do researchers balance reproducibility and innovation in scaling up synthesis?
- Answer : Adopt Quality by Design (QbD) principles:
- Critical Process Parameters (CPPs) : Temperature, reaction time.
- Critical Quality Attributes (CQAs) : Yield, purity.
Use design-of-experiments (DoE) software (e.g., MODDE) to optimize conditions. Contradictions between small- and large-scale batches often stem from heat/mass transfer inefficiencies; address via computational fluid dynamics (CFD) simulations .
Propriétés
IUPAC Name |
1-amino-3,7-dimethylpurine-2,6-dione | |
---|---|---|
Source | PubChem | |
URL | https://pubchem.ncbi.nlm.nih.gov | |
Description | Data deposited in or computed by PubChem | |
InChI |
InChI=1S/C7H9N5O2/c1-10-3-9-5-4(10)6(13)12(8)7(14)11(5)2/h3H,8H2,1-2H3 | |
Source | PubChem | |
URL | https://pubchem.ncbi.nlm.nih.gov | |
Description | Data deposited in or computed by PubChem | |
InChI Key |
NAVLIYUAMBPQRQ-UHFFFAOYSA-N | |
Source | PubChem | |
URL | https://pubchem.ncbi.nlm.nih.gov | |
Description | Data deposited in or computed by PubChem | |
Canonical SMILES |
CN1C=NC2=C1C(=O)N(C(=O)N2C)N | |
Source | PubChem | |
URL | https://pubchem.ncbi.nlm.nih.gov | |
Description | Data deposited in or computed by PubChem | |
Molecular Formula |
C7H9N5O2 | |
Source | PubChem | |
URL | https://pubchem.ncbi.nlm.nih.gov | |
Description | Data deposited in or computed by PubChem | |
DSSTOX Substance ID |
DTXSID30321018 | |
Record name | 1-Amino-3,7-dimethyl-3,7-dihydro-1H-purine-2,6-dione | |
Source | EPA DSSTox | |
URL | https://comptox.epa.gov/dashboard/DTXSID30321018 | |
Description | DSSTox provides a high quality public chemistry resource for supporting improved predictive toxicology. | |
Molecular Weight |
195.18 g/mol | |
Source | PubChem | |
URL | https://pubchem.ncbi.nlm.nih.gov | |
Description | Data deposited in or computed by PubChem | |
CAS No. |
81281-47-2 | |
Record name | NSC367963 | |
Source | DTP/NCI | |
URL | https://dtp.cancer.gov/dtpstandard/servlet/dwindex?searchtype=NSC&outputformat=html&searchlist=367963 | |
Description | The NCI Development Therapeutics Program (DTP) provides services and resources to the academic and private-sector research communities worldwide to facilitate the discovery and development of new cancer therapeutic agents. | |
Explanation | Unless otherwise indicated, all text within NCI products is free of copyright and may be reused without our permission. Credit the National Cancer Institute as the source. | |
Record name | 1-Amino-3,7-dimethyl-3,7-dihydro-1H-purine-2,6-dione | |
Source | EPA DSSTox | |
URL | https://comptox.epa.gov/dashboard/DTXSID30321018 | |
Description | DSSTox provides a high quality public chemistry resource for supporting improved predictive toxicology. | |
Retrosynthesis Analysis
AI-Powered Synthesis Planning: Our tool employs the Template_relevance Pistachio, Template_relevance Bkms_metabolic, Template_relevance Pistachio_ringbreaker, Template_relevance Reaxys, Template_relevance Reaxys_biocatalysis model, leveraging a vast database of chemical reactions to predict feasible synthetic routes.
One-Step Synthesis Focus: Specifically designed for one-step synthesis, it provides concise and direct routes for your target compounds, streamlining the synthesis process.
Accurate Predictions: Utilizing the extensive PISTACHIO, BKMS_METABOLIC, PISTACHIO_RINGBREAKER, REAXYS, REAXYS_BIOCATALYSIS database, our tool offers high-accuracy predictions, reflecting the latest in chemical research and data.
Strategy Settings
Precursor scoring | Relevance Heuristic |
---|---|
Min. plausibility | 0.01 |
Model | Template_relevance |
Template Set | Pistachio/Bkms_metabolic/Pistachio_ringbreaker/Reaxys/Reaxys_biocatalysis |
Top-N result to add to graph | 6 |
Feasible Synthetic Routes
Avertissement et informations sur les produits de recherche in vitro
Veuillez noter que tous les articles et informations sur les produits présentés sur BenchChem sont destinés uniquement à des fins informatives. Les produits disponibles à l'achat sur BenchChem sont spécifiquement conçus pour des études in vitro, qui sont réalisées en dehors des organismes vivants. Les études in vitro, dérivées du terme latin "in verre", impliquent des expériences réalisées dans des environnements de laboratoire contrôlés à l'aide de cellules ou de tissus. Il est important de noter que ces produits ne sont pas classés comme médicaments et n'ont pas reçu l'approbation de la FDA pour la prévention, le traitement ou la guérison de toute condition médicale, affection ou maladie. Nous devons souligner que toute forme d'introduction corporelle de ces produits chez les humains ou les animaux est strictement interdite par la loi. Il est essentiel de respecter ces directives pour assurer la conformité aux normes légales et éthiques en matière de recherche et d'expérimentation.