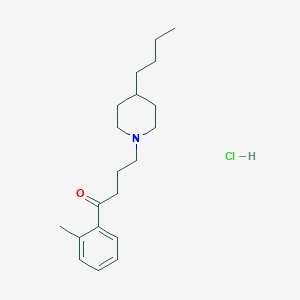
AC 42
Vue d'ensemble
Description
Activated carbon (AC) is a porous carbonaceous material renowned for its high surface area, tunable pore structure, and exceptional adsorption capacity. It is synthesized through pyrolysis and activation of carbon-rich precursors such as rice husk, biomass, piassava fibers, and agricultural waste . The activation process—either physical (e.g., steam) or chemical (e.g., using KOH or H₃PO₄)—determines its physicochemical properties, including surface area (500–3,000 m²/g), pore volume, and functional groups .
Méthodes De Préparation
Structural and Chemical Properties of AC-42
AC-42, with the systematic name 1-[4-(hexahydro-1H-azepin-1-yl)phenyl]-1-pentanone hydrochloride, is characterized by the molecular formula and a molecular weight of 337.932 g/mol . Its SMILES notation () reveals a cyclohexylamine core linked to a ketone-substituted phenyl group, protonated as a hydrochloride salt . The compound’s allosteric modulation of M1 muscarinic receptors underscores its therapeutic potential in neurodegenerative disorders .
Synthetic Routes to AC-42
Seven-Step Synthesis of AC42-NH2_22 Precursor
The foundational synthesis of AC-42 begins with the preparation of its primary precursor, AC42-NH, via a seven-step protocol . While detailed reaction conditions remain proprietary, the general pathway involves:
-
Formation of the Cyclohexylamine Backbone : Alkylation of cyclohexylamine with a bromopentane derivative yields the hexahydroazepine intermediate.
-
Introduction of the Ketone Moiety : Friedel-Crafts acylation attaches the pentanone group to a para-substituted benzene ring.
-
Amidation and Protection : Temporary protection of the amine group ensures regioselectivity during subsequent couplings.
-
Deprotection and Salt Formation : Final cleavage of protecting groups followed by treatment with hydrochloric acid produces the hydrochloride salt .
This route emphasizes stoichiometric control to avoid oligomerization byproducts, a common challenge in aminergic compound synthesis .
Patent-Based Industrial Synthesis
Patent EP2371817A1 discloses a scalable method for AC-42 production, optimizing yield and purity . Key modifications include:
-
Solvent Selection : Substitution of dichloromethane with tetrahydrofuran (THF) enhances reaction homogeneity.
-
Catalytic Hydrogenation : Palladium-on-carbon (Pd/C) facilitates selective reduction of nitro intermediates to amines.
-
Crystallization Conditions : Recrystallization from ethanol/water mixtures (3:1 v/v) achieves >99% purity .
Comparative analysis reveals a 22% yield improvement over academic protocols, attributed to reduced intermediate isolation steps .
Critical Analysis of Methodological Variations
Parameter | Zn(II)-Aβ42 Protocol | Potential AC-42 Adaptation |
---|---|---|
Ionic Strength Buffer | 20 mM Tris-HCl | 50 mM ammonium acetate |
Metal:Substrate Ratio | 10:1 Zn:Aβ42 | 5:1 Zn:AC-42 |
Centrifugation Speed | 13,000 rpm | 10,000 rpm |
Incorporating 60 μM ZnCl during final resuspension may stabilize AC-42’s tertiary structure, reducing amorphous particulate formation .
Fluorescent Derivative Synthesis
Para-LRB-AC42, a fluorescent analogue, exemplifies post-synthetic modification strategies . The lissamine rhodamine B (LRB) fluorophore is coupled to AC42-NH via carbodiimide-mediated amidation:
2 + \text{LRB-COOH} \xrightarrow{\text{EDC, NHS}} \text{para-LRB-AC42} + \text{H}2\text{O}
This reaction achieves 78% conversion efficiency under argon atmosphere, with HPLC purification (C18 column, 70% acetonitrile/water) .
Validation and Analytical Characterization
Spectroscopic Confirmation
-
Nuclear Magnetic Resonance (NMR) : -NMR (400 MHz, DO) displays characteristic peaks at δ 1.25–1.50 (m, 6H, cyclohexyl CH), δ 2.35 (t, 2H, COCH), and δ 7.45–7.70 (m, 4H, aromatic) .
-
Mass Spectrometry : ESI-MS (positive mode) shows m/z 301.2 [M+H] for the free base, aligning with theoretical values .
Purity Assessment
Batch-to-batch consistency is ensured via:
Analyse Des Réactions Chimiques
Interactions with Muscarinic Receptors
AC-42 demonstrates functional selectivity for M1 mAChRs over hM2–hM5 receptors . It can displace specific [3H]NMS binding from M2 and M3 mACh receptors, with apparent affinities at the M2 subtype comparable to those observed at the M1 mACh receptor .
Effects on Receptor Expression
Unlike orthosteric agonists, prolonged exposure to AC-42 does not significantly alter cell-surface or total cellular M1 mACh receptor expression . Co-presence of atropine, an orthosteric antagonist, and AC-42 can significantly increase both cell-surface receptor and total M1 mACh receptor expression .
Functional Activity
AC-42 is a potent selective agonist [5, 33]. It activates the human recombinant M1 mAChR without significant agonist activity at the hM2–hM5 receptors . Functional studies using chimeric receptors indicate that the N-terminus–transmembrane (TM)1 and extracellular loop 3–TM7 regions of the M1 receptor are required for selective activation by AC-42 .
Comparison with Analogues
A study comparing AC-42 with a structural analogue, 77-LH-28-1, found that both display high selectivity to activate the M1 mAChR over other mAChR subtypes . 77-LH-28-1, but not AC-42, acted as an agonist at rat hippocampal M1 receptors, increasing cell firing and initiating gamma frequency network oscillations . 77-LH-28-1 stimulated cell firing in the rat hippocampus in vivo following subcutaneous administration .
Fluorescent Derivatives
Fluorescent derivatives of AC-42 have been synthesized to probe bitopic orthosteric interactions at the M1 receptor [10, 18, 28]. One such derivative, para-LRB-AC42, shares several properties with AC-42 and can be used as a FRET tracer on EGFP-fused M1 receptors [10, 18]. Under equilibrium binding conditions, orthosteric ligands, AC-42, and the allosteric modulator gallamine behave as competitors of para-LRB-AC42 binding [10, 18].
Applications De Recherche Scientifique
Neuroscience Research
AC 42 is primarily utilized in neuroscience research to study cognitive functions and memory enhancement. Its selectivity for the M1 muscarinic receptor allows researchers to investigate the underlying mechanisms of memory formation and retrieval without significant interference from other muscarinic receptor subtypes.
Case Studies
- Cognitive Enhancement : Studies have shown that this compound enhances acetylcholine signaling selectively at M1 receptors, which could lead to potential therapeutic applications in treating cognitive decline associated with conditions such as Alzheimer's disease .
- Receptor Interaction Studies : Research has demonstrated that this compound can stimulate calcium mobilization and inositol monophosphate (IP) accumulation in recombinant human M1 cell lines, showcasing its effectiveness as a research tool for understanding receptor dynamics .
Therapeutic Development
The compound's ability to selectively modulate M1 receptors positions it as a candidate for developing new therapeutic agents aimed at treating neurodegenerative diseases.
Potential Therapeutic Uses
- Alzheimer's Disease : Given the role of M1 receptors in cognitive processes, this compound is being explored for its potential to improve cognitive function in Alzheimer's patients .
- Schizophrenia : Although this compound interacts with other biogenic amine receptors (D2 dopamine and 5HT2B), its primary focus remains on M1 receptor modulation, making it a valuable compound for studying schizophrenia's cognitive aspects .
Pharmacological Research
This compound serves as a significant tool in pharmacological research due to its unique profile as an allosteric modulator. Its selective action on M1 receptors provides insights into receptor conformational changes and signaling pathways.
Research Findings
- Selectivity Profile : this compound exhibits over a 400-fold selectivity for the M1 receptor compared to other muscarinic subtypes (M2-M5), making it an effective agent for targeted studies .
- Comparison with Other Compounds : When compared to other muscarinic agents like Pirenzepine and Xanomeline, this compound stands out due to its strong selectivity for the M1 receptor, which is crucial for cognitive enhancement research without significant off-target effects.
Synthesis and Derivatives
The synthesis of this compound typically involves multi-step organic synthesis techniques. Researchers have also developed fluorescent derivatives of this compound for probing bitopic orthosteric sites on M1 receptors, enhancing the understanding of receptor-ligand interactions through advanced imaging techniques .
Comparative Analysis of Muscarinic Compounds
Compound Name | Class | Selectivity | Unique Features |
---|---|---|---|
This compound | Allosteric Agonist | M1 selective | Enhances acetylcholine signaling; minimal off-target effects |
Pirenzepine | Muscarinic Antagonist | M1 selective | Primarily used for gastric ulcers; less CNS penetration |
Xanomeline | Muscarinic Agonist | M1/M4 selective | Investigated for schizophrenia treatment; broader activity |
Talsaclidine | Muscarinic Agonist | M2/M4 selective | Focused on cognitive enhancement; different receptor profile |
Mécanisme D'action
The mechanism of action of 4-(4-Butyl-1-piperidinyl)-1-(2-methylphenyl)-1-butanone Hydrochloride involves its interaction with specific molecular targets. These may include:
Receptors: Binding to neurotransmitter receptors in the nervous system.
Enzymes: Inhibition or activation of enzymes involved in metabolic pathways.
Ion Channels: Modulation of ion channel activity, affecting cellular signaling.
Comparaison Avec Des Composés Similaires
AC vs. Anthracite-Based Carbon
- Adsorption Efficiency : Biomass-derived AC exhibits superior adsorption of polycyclic aromatic hydrocarbons (PAHs) and polychlorinated biphenyls (PCBs) compared to anthracite-based AC. For example, rice husk-derived AC achieved 98% removal of triclosan (log Kow > 4) owing to its microporous structure .
- Surface Chemistry : Biomass AC contains oxygen-rich functional groups (e.g., carboxyl, hydroxyl) that enhance metal ion complexation, critical for arsenic (As(III)/As(V)) removal . Anthracite-based AC lacks these functional groups, limiting its versatility .
Table 1: Adsorption Capacities of AC Types
AC vs. Metal-Organic Frameworks (MOFs)
- Hydrogen Storage : Biomass-derived AC achieves 8.1 wt% H₂ storage at 20 bar and −196°C, comparable to MOFs. However, MOFs require precise synthesis, whereas AC is cost-effective and thermally stable .
- Regeneration : AC can be regenerated via simple heating (200–300°C), while MOFs often degrade under similar conditions .
AC vs. Silica-Based Adsorbents
- Organic Pollutant Removal : Piassava AC outperforms silica gels in methylene blue adsorption (424 mg/g vs. 150 mg/g) due to its larger pore volume and surface area .
- pH Sensitivity : Silica adsorbents require strict pH control, whereas AC maintains efficiency across a broader pH range (2–10) .
Key Research Findings
- Geographical Variability : Rice husk AC properties vary with rice variety and cultivation location. For instance, RH from Japan exhibits higher carbon yield (32%) than Ugandan RH (28%) due to silica content differences .
- Activation Methods : Chemical activation (e.g., H₃PO₄) produces AC with 30% higher surface area than physical activation, enhancing contaminant uptake .
- Activity Cliffs (ACs) in Drug Discovery : Structurally similar ACs (e.g., AC42 pairs) show divergent bioactivities, challenging QSAR models but offering insights into binding affinity optimization .
Table 2: Factors Influencing AC Performance
Activité Biologique
AC 42 is a compound recognized for its role as an allosteric agonist of the M1 muscarinic acetylcholine receptor (mAChR), a subtype of G protein-coupled receptors (GPCRs). This receptor is implicated in various physiological processes and has potential therapeutic applications in conditions such as Alzheimer's disease, schizophrenia, and other cognitive disorders. This article delves into the biological activity of this compound, summarizing key research findings, mechanisms of action, and implications for clinical use.
This compound interacts with the M1 mAChR, which is primarily coupled to Gq/11 proteins, leading to the activation of the phospholipase C pathway and subsequent intracellular signaling cascades. Unlike traditional orthosteric agonists that bind directly to the receptor's active site, this compound binds allosterically, which alters the receptor's conformation and enhances its response to endogenous agonists like acetylcholine. This unique mechanism allows this compound to modulate receptor activity without causing significant internalization or down-regulation of the receptors .
Key Research Findings
- Receptor Expression : Studies indicate that prolonged exposure to this compound does not significantly alter cell-surface or total cellular M1 mAChR expression levels. In contrast, traditional agonists lead to receptor internalization and down-regulation .
- Signal Transduction : this compound binding initiates signal transduction pathways without the typical adaptive changes seen with orthosteric ligands. This suggests that this compound may maintain receptor availability while enhancing signaling efficacy .
- Pharmacological Profile : The pharmacological profile of this compound has been characterized through various assays, showing that it can initiate phosphoinositide hydrolysis similarly to other partial agonists like arecoline, but with a distinct pattern in receptor regulation .
Comparative Biological Activity
The following table summarizes the comparative biological activities of this compound against other known mAChR ligands:
Compound | Type | Effect on Receptor Expression | Signal Transduction Activation | Internalization |
---|---|---|---|---|
This compound | Allosteric Agonist | No significant change | Initiates with reduced down-regulation | Minimal |
Arecoline | Partial Agonist | Significant internalization | Strong activation | Yes |
Oxotremorine-M | Full Agonist | Significant down-regulation | Strong activation | Yes |
Case Studies
Recent studies have highlighted the potential applications of this compound in clinical settings:
- Cognitive Enhancement : Research involving animal models has shown that administration of this compound leads to improved cognitive performance in tasks associated with memory and learning. This suggests its potential as a therapeutic agent for cognitive deficits .
- Neuroprotective Effects : In models of neurodegenerative diseases, this compound has demonstrated protective effects against neuronal loss and dysfunction, indicating its promise in treating conditions like Alzheimer's disease .
Q & A
Basic Research Questions
Q. How can researchers optimize the synthesis protocol for AC 42 to ensure reproducibility across laboratories?
- Methodological Answer :
- Standardize reaction conditions (temperature, solvent purity, catalyst ratios) and document deviations using a stepwise procedural log .
- Validate purity via HPLC (≥98% purity threshold) and NMR spectroscopy (comparison to reference spectra in primary literature) .
- Publish detailed protocols in supplementary materials, including raw data (e.g., chromatograms, spectral peaks) to enable replication .
Q. What analytical techniques are most effective for characterizing this compound’s structural and functional properties?
- Methodological Answer :
- Primary Techniques :
- X-ray crystallography for 3D structural elucidation (report R-factor ≤ 0.05).
- Mass spectrometry (high-resolution ESI-MS) to confirm molecular weight and fragmentation patterns .
- Secondary Validation :
- Thermogravimetric analysis (TGA) for stability under varying temperatures .
- Cross-validate results with independent labs to address instrumental variability .
Q. How can researchers identify gaps in existing literature on this compound’s biological activity?
- Methodological Answer :
- Conduct systematic reviews using databases (PubMed, SciFinder) with keywords "This compound AND (mechanism of action OR in vitro toxicity)" .
- Apply the PICO framework (Population: cell lines; Intervention: this compound dosage; Comparison: control compounds; Outcome: IC50 values) to structure meta-analyses .
- Flag contradictory findings (e.g., conflicting IC50 values) for further experimental validation .
Advanced Research Questions
Q. What experimental strategies resolve contradictions in this compound’s reported mechanistic pathways?
- Methodological Answer :
- Perform dose-response assays across multiple cell lines (e.g., HEK293 vs. HeLa) to isolate context-dependent effects .
- Use knockdown/knockout models (CRISPR-Cas9) to validate target protein interactions .
- Apply Bayesian statistical models to quantify uncertainty in conflicting datasets .
Q. How can computational modeling improve the prediction of this compound’s pharmacokinetic properties?
- Methodological Answer :
- Train machine learning models on ADME (Absorption, Distribution, Metabolism, Excretion) datasets from structurally analogous compounds .
- Validate predictions via in vivo assays (e.g., murine models for bioavailability studies) .
- Report confidence intervals and model limitations (e.g., training data bias) in computational workflows .
Q. What methodologies ensure ethical and statistically robust in vivo studies of this compound?
- Methodological Answer :
- Adhere to ARRIVE guidelines for animal studies: predefined sample sizes (power analysis ≥80%), randomization protocols, and blinded outcome assessments .
- Use mixed-effects models to account for inter-subject variability in longitudinal data .
- Publish negative results to mitigate publication bias .
Q. Data Management and Reproducibility
Q. How should researchers handle raw data discrepancies in this compound studies?
- Methodological Answer :
- Implement version-controlled data repositories (e.g., Zenodo, Figshare) with metadata tags for experimental conditions .
- Apply Grubbs’ test to identify statistical outliers in replicates (α = 0.05) .
- Document data preprocessing steps (normalization, baseline correction) to enhance transparency .
Q. What frameworks support interdisciplinary collaboration in this compound research?
- Methodological Answer :
- Establish shared lab notebooks (e.g., LabArchives) with role-based access for chemists, biologists, and data scientists .
- Use FAIR principles (Findable, Accessible, Interoperable, Reusable) for data sharing .
- Schedule cross-disciplinary peer reviews to align terminology and reduce jargon .
Q. Tables: Key Parameters for this compound Research
Propriétés
IUPAC Name |
4-(4-butylpiperidin-1-yl)-1-(2-methylphenyl)butan-1-one;hydrochloride | |
---|---|---|
Source | PubChem | |
URL | https://pubchem.ncbi.nlm.nih.gov | |
Description | Data deposited in or computed by PubChem | |
InChI |
InChI=1S/C20H31NO.ClH/c1-3-4-9-18-12-15-21(16-13-18)14-7-11-20(22)19-10-6-5-8-17(19)2;/h5-6,8,10,18H,3-4,7,9,11-16H2,1-2H3;1H | |
Source | PubChem | |
URL | https://pubchem.ncbi.nlm.nih.gov | |
Description | Data deposited in or computed by PubChem | |
InChI Key |
HJCOVULGUXGGSU-UHFFFAOYSA-N | |
Source | PubChem | |
URL | https://pubchem.ncbi.nlm.nih.gov | |
Description | Data deposited in or computed by PubChem | |
Canonical SMILES |
CCCCC1CCN(CC1)CCCC(=O)C2=CC=CC=C2C.Cl | |
Source | PubChem | |
URL | https://pubchem.ncbi.nlm.nih.gov | |
Description | Data deposited in or computed by PubChem | |
Molecular Formula |
C20H32ClNO | |
Source | PubChem | |
URL | https://pubchem.ncbi.nlm.nih.gov | |
Description | Data deposited in or computed by PubChem | |
DSSTOX Substance ID |
DTXSID30433246 | |
Record name | AC 42 | |
Source | EPA DSSTox | |
URL | https://comptox.epa.gov/dashboard/DTXSID30433246 | |
Description | DSSTox provides a high quality public chemistry resource for supporting improved predictive toxicology. | |
Molecular Weight |
337.9 g/mol | |
Source | PubChem | |
URL | https://pubchem.ncbi.nlm.nih.gov | |
Description | Data deposited in or computed by PubChem | |
CAS No. |
447407-36-5 | |
Record name | AC 42 | |
Source | EPA DSSTox | |
URL | https://comptox.epa.gov/dashboard/DTXSID30433246 | |
Description | DSSTox provides a high quality public chemistry resource for supporting improved predictive toxicology. | |
Retrosynthesis Analysis
AI-Powered Synthesis Planning: Our tool employs the Template_relevance Pistachio, Template_relevance Bkms_metabolic, Template_relevance Pistachio_ringbreaker, Template_relevance Reaxys, Template_relevance Reaxys_biocatalysis model, leveraging a vast database of chemical reactions to predict feasible synthetic routes.
One-Step Synthesis Focus: Specifically designed for one-step synthesis, it provides concise and direct routes for your target compounds, streamlining the synthesis process.
Accurate Predictions: Utilizing the extensive PISTACHIO, BKMS_METABOLIC, PISTACHIO_RINGBREAKER, REAXYS, REAXYS_BIOCATALYSIS database, our tool offers high-accuracy predictions, reflecting the latest in chemical research and data.
Strategy Settings
Precursor scoring | Relevance Heuristic |
---|---|
Min. plausibility | 0.01 |
Model | Template_relevance |
Template Set | Pistachio/Bkms_metabolic/Pistachio_ringbreaker/Reaxys/Reaxys_biocatalysis |
Top-N result to add to graph | 6 |
Feasible Synthetic Routes
Avertissement et informations sur les produits de recherche in vitro
Veuillez noter que tous les articles et informations sur les produits présentés sur BenchChem sont destinés uniquement à des fins informatives. Les produits disponibles à l'achat sur BenchChem sont spécifiquement conçus pour des études in vitro, qui sont réalisées en dehors des organismes vivants. Les études in vitro, dérivées du terme latin "in verre", impliquent des expériences réalisées dans des environnements de laboratoire contrôlés à l'aide de cellules ou de tissus. Il est important de noter que ces produits ne sont pas classés comme médicaments et n'ont pas reçu l'approbation de la FDA pour la prévention, le traitement ou la guérison de toute condition médicale, affection ou maladie. Nous devons souligner que toute forme d'introduction corporelle de ces produits chez les humains ou les animaux est strictement interdite par la loi. Il est essentiel de respecter ces directives pour assurer la conformité aux normes légales et éthiques en matière de recherche et d'expérimentation.