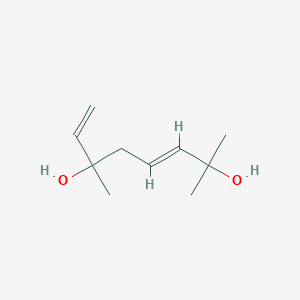
2,6-Diméthyl-3,7-octadiène-2,6-diol
Vue d'ensemble
Description
2,6-Dimethyl-3,7-octadiene-2,6-diol is an organic compound with the molecular formula C10H18O2. It is also known by other names such as trans-3,7-dimethyl-1,5-octadiene-3,7-diol and (E)-2,6-dimethyl-3,7-octadiene-2,6-diol . This compound is a colorless to pale yellow liquid that is soluble in various organic solvents like ethanol and ether . It is a monoterpenoid found naturally in the fruits of Gardenia jasminoides Ellis .
Applications De Recherche Scientifique
2,6-Dimethyl-3,7-octadiene-2,6-diol has a wide range of scientific research applications. In chemistry, it is used as an intermediate in the synthesis of other organic compounds . In biology, it is studied for its potential role in plant metabolism and its presence in various plant species . In medicine, it is explored for its potential therapeutic properties, including its use in the synthesis of pharmaceuticals . Industrially, it is used in the production of fragrances and flavorings due to its pleasant aroma .
Safety and Hazards
2,6-Dimethyl-3,7-octadiene-2,6-diol may cause irritation to the eyes and skin . Therefore, appropriate personal protective measures, such as wearing gloves, glasses, and protective clothing, should be taken when handling this compound . It should be stored and used away from sources of fire and high temperatures .
Méthodes De Préparation
2,6-Dimethyl-3,7-octadiene-2,6-diol can be synthesized through several methods. One common synthetic route involves the hydroxylation reaction using hydrogen gas and a palladium chloride catalyst . This method is widely used in industrial production due to its efficiency and cost-effectiveness. The reaction conditions typically involve moderate temperatures and pressures to ensure optimal yield and purity of the product .
Analyse Des Réactions Chimiques
2,6-Dimethyl-3,7-octadiene-2,6-diol undergoes various chemical reactions, including oxidation, reduction, and substitution reactions. Common reagents used in these reactions include oxidizing agents like potassium permanganate and reducing agents like lithium aluminum hydride . The major products formed from these reactions depend on the specific conditions and reagents used. For example, oxidation of 2,6-dimethyl-3,7-octadiene-2,6-diol can lead to the formation of corresponding ketones or carboxylic acids .
Mécanisme D'action
The mechanism of action of 2,6-dimethyl-3,7-octadiene-2,6-diol involves its interaction with specific molecular targets and pathways. As a monoterpenoid, it can interact with enzymes and receptors in biological systems, influencing various metabolic processes . The exact molecular targets and pathways involved depend on the specific context and application of the compound .
Comparaison Avec Des Composés Similaires
2,6-Dimethyl-3,7-octadiene-2,6-diol can be compared with other similar compounds such as 3,7-dimethylocta-1,5-dien-3,7-diol (Terpenediol I) and 2,6-dimethylocta-3,7-diene-2,6-diol . These compounds share similar structural features and chemical properties but differ in their specific functional groups and stereochemistry . The uniqueness of 2,6-dimethyl-3,7-octadiene-2,6-diol lies in its specific arrangement of methyl and hydroxyl groups, which confer distinct chemical reactivity and biological activity .
Propriétés
IUPAC Name |
(3E)-2,6-dimethylocta-3,7-diene-2,6-diol | |
---|---|---|
Source | PubChem | |
URL | https://pubchem.ncbi.nlm.nih.gov | |
Description | Data deposited in or computed by PubChem | |
InChI |
InChI=1S/C10H18O2/c1-5-10(4,12)8-6-7-9(2,3)11/h5-7,11-12H,1,8H2,2-4H3/b7-6+ | |
Source | PubChem | |
URL | https://pubchem.ncbi.nlm.nih.gov | |
Description | Data deposited in or computed by PubChem | |
InChI Key |
QEOHJVNDENHRCH-VOTSOKGWSA-N | |
Source | PubChem | |
URL | https://pubchem.ncbi.nlm.nih.gov | |
Description | Data deposited in or computed by PubChem | |
Canonical SMILES |
CC(C)(C=CCC(C)(C=C)O)O | |
Source | PubChem | |
URL | https://pubchem.ncbi.nlm.nih.gov | |
Description | Data deposited in or computed by PubChem | |
Isomeric SMILES |
CC(C)(/C=C/CC(C)(C=C)O)O | |
Source | PubChem | |
URL | https://pubchem.ncbi.nlm.nih.gov | |
Description | Data deposited in or computed by PubChem | |
Molecular Formula |
C10H18O2 | |
Source | PubChem | |
URL | https://pubchem.ncbi.nlm.nih.gov | |
Description | Data deposited in or computed by PubChem | |
Molecular Weight |
170.25 g/mol | |
Source | PubChem | |
URL | https://pubchem.ncbi.nlm.nih.gov | |
Description | Data deposited in or computed by PubChem | |
CAS No. |
13741-21-4 | |
Record name | 2,6-Dimethyl-3,7-octadiene-2,6-diol | |
Source | Human Metabolome Database (HMDB) | |
URL | http://www.hmdb.ca/metabolites/HMDB0036990 | |
Description | The Human Metabolome Database (HMDB) is a freely available electronic database containing detailed information about small molecule metabolites found in the human body. | |
Explanation | HMDB is offered to the public as a freely available resource. Use and re-distribution of the data, in whole or in part, for commercial purposes requires explicit permission of the authors and explicit acknowledgment of the source material (HMDB) and the original publication (see the HMDB citing page). We ask that users who download significant portions of the database cite the HMDB paper in any resulting publications. | |
Retrosynthesis Analysis
AI-Powered Synthesis Planning: Our tool employs the Template_relevance Pistachio, Template_relevance Bkms_metabolic, Template_relevance Pistachio_ringbreaker, Template_relevance Reaxys, Template_relevance Reaxys_biocatalysis model, leveraging a vast database of chemical reactions to predict feasible synthetic routes.
One-Step Synthesis Focus: Specifically designed for one-step synthesis, it provides concise and direct routes for your target compounds, streamlining the synthesis process.
Accurate Predictions: Utilizing the extensive PISTACHIO, BKMS_METABOLIC, PISTACHIO_RINGBREAKER, REAXYS, REAXYS_BIOCATALYSIS database, our tool offers high-accuracy predictions, reflecting the latest in chemical research and data.
Strategy Settings
Precursor scoring | Relevance Heuristic |
---|---|
Min. plausibility | 0.01 |
Model | Template_relevance |
Template Set | Pistachio/Bkms_metabolic/Pistachio_ringbreaker/Reaxys/Reaxys_biocatalysis |
Top-N result to add to graph | 6 |
Feasible Synthetic Routes
Q1: What is the significance of 2,6-Dimethyl-3,7-octadiene-2,6-diol in tea aroma?
A: Research suggests that 2,6-Dimethyl-3,7-octadiene-2,6-diol plays a crucial role in the unique aroma of Darjeeling tea. It is believed to be a precursor to 3,7-dimethyl-1,5,7-octatrien-3-ol (hotrienol), a key aroma compound. This connection was observed in studies comparing the volatile components of different tea types, including Darjeeling and Chan Pin oolong, which share a similar aroma profile. Notably, Chan Pin oolong, produced from tea leaves infested with green flies (Emposca flavescens) similar to Darjeeling production, exhibited high levels of 2,6-Dimethyl-3,7-octadiene-2,6-diol. This finding further supports the compound's role in shaping the characteristic Darjeeling tea aroma.
Q2: Is 2,6-Dimethyl-3,7-octadiene-2,6-diol found in other natural sources besides tea?
A: Yes, 2,6-Dimethyl-3,7-octadiene-2,6-diol has been identified in various natural sources. It is a prominent component in leatherwood (Eucryphia lucida) honey, originating from the dehydration of the diol present in the leatherwood plant. Additionally, it exists in a glycosidically bound form in lulo fruit (Solanum vestissimum D.) peelings. Its presence has also been confirmed in apricots (Prunus armeniaca var. ansu Max.) as a glycosidically bound volatile.
Q3: How does 2,6-Dimethyl-3,7-octadiene-2,6-diol contribute to attracting predatory spiders?
A: Studies investigating the interaction between tea plants, green leafhoppers (Empoasca vitis), and predatory spiders (Evarcha albaria) revealed the role of 2,6-Dimethyl-3,7-octadiene-2,6-diol in attracting the spiders. Specifically, when tea shoots are damaged by E. vitis, they release a blend of volatiles, including 2,6-Dimethyl-3,7-octadiene-2,6-diol and indole, that significantly attracts E. albaria. These findings suggest that the compound acts as a signaling molecule, attracting the predatory spiders to the damaged tea plants.
Q4: What are the structural characteristics of 2,6-Dimethyl-3,7-octadiene-2,6-diol?
A4: While the provided abstracts do not offer specific spectroscopic data, we can deduce some structural information. As the name suggests, 2,6-Dimethyl-3,7-octadiene-2,6-diol possesses:
Q5: What analytical techniques are used to identify and quantify 2,6-Dimethyl-3,7-octadiene-2,6-diol?
A: Several analytical techniques are employed to study 2,6-Dimethyl-3,7-octadiene-2,6-diol. Gas chromatography (GC) coupled with mass spectrometry (MS) is a commonly used method, enabling the separation and identification of volatile compounds in complex mixtures. Additionally, nuclear magnetic resonance (NMR) spectroscopy is utilized for structural elucidation, particularly in identifying the compound's isomers and derivatives. These techniques are essential for characterizing and quantifying 2,6-Dimethyl-3,7-octadiene-2,6-diol in various matrices.
Avertissement et informations sur les produits de recherche in vitro
Veuillez noter que tous les articles et informations sur les produits présentés sur BenchChem sont destinés uniquement à des fins informatives. Les produits disponibles à l'achat sur BenchChem sont spécifiquement conçus pour des études in vitro, qui sont réalisées en dehors des organismes vivants. Les études in vitro, dérivées du terme latin "in verre", impliquent des expériences réalisées dans des environnements de laboratoire contrôlés à l'aide de cellules ou de tissus. Il est important de noter que ces produits ne sont pas classés comme médicaments et n'ont pas reçu l'approbation de la FDA pour la prévention, le traitement ou la guérison de toute condition médicale, affection ou maladie. Nous devons souligner que toute forme d'introduction corporelle de ces produits chez les humains ou les animaux est strictement interdite par la loi. Il est essentiel de respecter ces directives pour assurer la conformité aux normes légales et éthiques en matière de recherche et d'expérimentation.