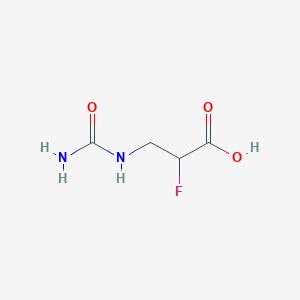
alpha-Fluoro-beta-ureidopropionic acid
Vue d'ensemble
Description
Barnidipine (chlorhydrate) est un bloqueur des canaux calciques à action prolongée appartenant au groupe des dihydropyridines. Il est principalement utilisé dans le traitement de l'hypertension. La barnidipine présente une forte affinité pour les canaux calciques dans les cellules musculaires lisses de la paroi vasculaire, ce qui entraîne une vasodilatation et une diminution de la pression artérielle .
Méthodes De Préparation
Voies de synthèse et conditions de réaction : La synthèse de la barnidipine (chlorhydrate) implique plusieurs étapes :
Réaction du 3-hydroxypropionitrile avec le dimère de cétène : pour obtenir un composé intermédiaire.
Réaction de l'intermédiaire avec le m-nitrobenzaldéhyde : pour former un autre intermédiaire.
Réaction avec le bêta-amino éthyl crotonate : pour produire un autre intermédiaire.
Hydrolyse par une base alcaline : pour obtenir un composé.
Résolution avec une base organique chirale : pour isoler l'énantiomère souhaité.
Réaction avec l'alcool benzyl pyrrole : pour former le composé final.
Addition du composé final à une solution d'acide chlorhydrique : pour obtenir la barnidipine (chlorhydrate).
Méthodes de production industrielle : La production industrielle de la barnidipine (chlorhydrate) suit la même voie de synthèse, mais elle est optimisée pour obtenir des rendements et une pureté plus élevés. Le procédé implique des conditions de réaction douces, des produits facilement séparables et purifiable, et l'utilisation de matières premières facilement disponibles .
Analyse Des Réactions Chimiques
Types de réactions : La barnidipine (chlorhydrate) subit diverses réactions chimiques, notamment :
Oxydation : Implique l'ajout d'oxygène ou l'élimination d'hydrogène.
Réduction : Implique l'ajout d'hydrogène ou l'élimination d'oxygène.
Substitution : Implique le remplacement d'un groupe fonctionnel par un autre.
Réactifs et conditions courants :
Oxydation : Les réactifs courants comprennent le permanganate de potassium et le peroxyde d'hydrogène.
Réduction : Les réactifs courants comprennent l'hydrure de lithium et d'aluminium et le borohydrure de sodium.
Substitution : Les réactifs courants comprennent les halogènes et les nucléophiles.
Produits principaux : Les produits principaux formés par ces réactions dépendent des réactifs et des conditions spécifiques utilisés. Par exemple, l'oxydation peut produire des acides carboxyliques, tandis que la réduction peut produire des alcools .
4. Applications de la recherche scientifique
La barnidipine (chlorhydrate) a plusieurs applications de recherche scientifique :
Chimie : Utilisé comme composé modèle dans l'étude des bloqueurs des canaux calciques.
Biologie : Étudié pour ses effets sur les canaux calciques cellulaires et les cellules musculaires lisses vasculaires.
Médecine : Utilisé dans les essais cliniques pour le traitement de l'hypertension et des maladies cardiovasculaires associées.
Industrie : Employé dans le développement de nouveaux médicaments antihypertenseurs et formulations
5. Mécanisme d'action
La barnidipine (chlorhydrate) exerce ses effets en bloquant les canaux calciques de type L dans les cellules musculaires lisses de la paroi vasculaire. Cette inhibition empêche les ions calcium d'entrer dans les cellules, ce qui entraîne la relaxation des muscles lisses et la vasodilatation. La réduction de la résistance vasculaire périphérique entraîne une baisse de la pression artérielle .
Applications De Recherche Scientifique
Barnidipine (hydrochloride) has several scientific research applications:
Chemistry: Used as a model compound in the study of calcium channel blockers.
Biology: Investigated for its effects on cellular calcium channels and vascular smooth muscle cells.
Medicine: Used in clinical trials for the treatment of hypertension and related cardiovascular conditions.
Industry: Employed in the development of new antihypertensive drugs and formulations
Mécanisme D'action
Barnidipine (hydrochloride) exerts its effects by blocking L-type calcium channels in the smooth muscle cells of the vascular wall. This inhibition prevents calcium ions from entering the cells, leading to relaxation of the smooth muscle and vasodilation. The reduction in peripheral vascular resistance results in lowered blood pressure .
Comparaison Avec Des Composés Similaires
La barnidipine (chlorhydrate) est comparée à d'autres bloqueurs des canaux calciques tels que l'amlodipine, la nitrendipine, l'aténolol, l'énalapril et l'hydrochlorothiazide. Contrairement à ces composés, la barnidipine est constituée d'un seul énantiomère, ce qui offre une sélectivité pharmacologique plus élevée et moins d'effets secondaires . Des composés similaires comprennent :
- Amlodipine
- Nitrendipine
- Atenolol
- Enalapril
- Hydrochlorothiazide
La composition unique de la barnidipine en un seul énantiomère lui confère un avantage distinct en termes d'efficacité et de sécurité .
Propriétés
IUPAC Name |
3-(carbamoylamino)-2-fluoropropanoic acid | |
---|---|---|
Source | PubChem | |
URL | https://pubchem.ncbi.nlm.nih.gov | |
Description | Data deposited in or computed by PubChem | |
InChI |
InChI=1S/C4H7FN2O3/c5-2(3(8)9)1-7-4(6)10/h2H,1H2,(H,8,9)(H3,6,7,10) | |
Source | PubChem | |
URL | https://pubchem.ncbi.nlm.nih.gov | |
Description | Data deposited in or computed by PubChem | |
InChI Key |
FKTHAKABFGARQH-UHFFFAOYSA-N | |
Source | PubChem | |
URL | https://pubchem.ncbi.nlm.nih.gov | |
Description | Data deposited in or computed by PubChem | |
Canonical SMILES |
C(C(C(=O)O)F)NC(=O)N | |
Source | PubChem | |
URL | https://pubchem.ncbi.nlm.nih.gov | |
Description | Data deposited in or computed by PubChem | |
Molecular Formula |
C4H7FN2O3 | |
Source | PubChem | |
URL | https://pubchem.ncbi.nlm.nih.gov | |
Description | Data deposited in or computed by PubChem | |
DSSTOX Substance ID |
DTXSID50964507 | |
Record name | 2-Fluoro-3-{[hydroxy(imino)methyl]amino}propanoic acid | |
Source | EPA DSSTox | |
URL | https://comptox.epa.gov/dashboard/DTXSID50964507 | |
Description | DSSTox provides a high quality public chemistry resource for supporting improved predictive toxicology. | |
Molecular Weight |
150.11 g/mol | |
Source | PubChem | |
URL | https://pubchem.ncbi.nlm.nih.gov | |
Description | Data deposited in or computed by PubChem | |
CAS No. |
5006-64-4 | |
Record name | α-Fluoro-β-ureidopropionic acid | |
Source | CAS Common Chemistry | |
URL | https://commonchemistry.cas.org/detail?cas_rn=5006-64-4 | |
Description | CAS Common Chemistry is an open community resource for accessing chemical information. Nearly 500,000 chemical substances from CAS REGISTRY cover areas of community interest, including common and frequently regulated chemicals, and those relevant to high school and undergraduate chemistry classes. This chemical information, curated by our expert scientists, is provided in alignment with our mission as a division of the American Chemical Society. | |
Explanation | The data from CAS Common Chemistry is provided under a CC-BY-NC 4.0 license, unless otherwise stated. | |
Record name | alpha-Fluoro-beta-ureidopropionic acid | |
Source | ChemIDplus | |
URL | https://pubchem.ncbi.nlm.nih.gov/substance/?source=chemidplus&sourceid=0005006644 | |
Description | ChemIDplus is a free, web search system that provides access to the structure and nomenclature authority files used for the identification of chemical substances cited in National Library of Medicine (NLM) databases, including the TOXNET system. | |
Record name | 2-Fluoro-3-{[hydroxy(imino)methyl]amino}propanoic acid | |
Source | EPA DSSTox | |
URL | https://comptox.epa.gov/dashboard/DTXSID50964507 | |
Description | DSSTox provides a high quality public chemistry resource for supporting improved predictive toxicology. | |
Record name | 3-[(Aminocarbonyl)amino]-2-fluoro-propanoic Acid | |
Source | European Chemicals Agency (ECHA) | |
URL | https://echa.europa.eu/information-on-chemicals | |
Description | The European Chemicals Agency (ECHA) is an agency of the European Union which is the driving force among regulatory authorities in implementing the EU's groundbreaking chemicals legislation for the benefit of human health and the environment as well as for innovation and competitiveness. | |
Explanation | Use of the information, documents and data from the ECHA website is subject to the terms and conditions of this Legal Notice, and subject to other binding limitations provided for under applicable law, the information, documents and data made available on the ECHA website may be reproduced, distributed and/or used, totally or in part, for non-commercial purposes provided that ECHA is acknowledged as the source: "Source: European Chemicals Agency, http://echa.europa.eu/". Such acknowledgement must be included in each copy of the material. ECHA permits and encourages organisations and individuals to create links to the ECHA website under the following cumulative conditions: Links can only be made to webpages that provide a link to the Legal Notice page. | |
Record name | .ALPHA.-FLUOROURIDOPROPIONIC ACID | |
Source | FDA Global Substance Registration System (GSRS) | |
URL | https://gsrs.ncats.nih.gov/ginas/app/beta/substances/0BXG1I9G0W | |
Description | The FDA Global Substance Registration System (GSRS) enables the efficient and accurate exchange of information on what substances are in regulated products. Instead of relying on names, which vary across regulatory domains, countries, and regions, the GSRS knowledge base makes it possible for substances to be defined by standardized, scientific descriptions. | |
Explanation | Unless otherwise noted, the contents of the FDA website (www.fda.gov), both text and graphics, are not copyrighted. They are in the public domain and may be republished, reprinted and otherwise used freely by anyone without the need to obtain permission from FDA. Credit to the U.S. Food and Drug Administration as the source is appreciated but not required. | |
Retrosynthesis Analysis
AI-Powered Synthesis Planning: Our tool employs the Template_relevance Pistachio, Template_relevance Bkms_metabolic, Template_relevance Pistachio_ringbreaker, Template_relevance Reaxys, Template_relevance Reaxys_biocatalysis model, leveraging a vast database of chemical reactions to predict feasible synthetic routes.
One-Step Synthesis Focus: Specifically designed for one-step synthesis, it provides concise and direct routes for your target compounds, streamlining the synthesis process.
Accurate Predictions: Utilizing the extensive PISTACHIO, BKMS_METABOLIC, PISTACHIO_RINGBREAKER, REAXYS, REAXYS_BIOCATALYSIS database, our tool offers high-accuracy predictions, reflecting the latest in chemical research and data.
Strategy Settings
Precursor scoring | Relevance Heuristic |
---|---|
Min. plausibility | 0.01 |
Model | Template_relevance |
Template Set | Pistachio/Bkms_metabolic/Pistachio_ringbreaker/Reaxys/Reaxys_biocatalysis |
Top-N result to add to graph | 6 |
Feasible Synthetic Routes
Q1: How is alpha-Fluoro-beta-ureidopropionic acid formed in the body?
A: this compound is formed as a metabolite during the breakdown of 5-fluorouracil (5-FU). Following the administration of 5-FU, it is rapidly metabolized, with a significant portion undergoing catabolism. The enzyme dihydropyrimidine dehydrogenase (DPD) catalyzes the first step in this catabolic pathway, converting 5-FU into dihydrofluorouracil (FUH2). FUH2 is further metabolized into this compound (FUPA) [, ].
Q2: What is the significance of this compound formation in 5-FU therapy?
A: The formation of FUPA signifies the breakdown and inactivation of 5-FU in the body. This catabolic pathway significantly reduces the amount of 5-FU available to exert its antitumor effects [, ]. Therefore, strategies to modulate 5-FU catabolism, such as using DPD inhibitors like 5-ethynyluracil (EU), are being investigated to enhance the therapeutic efficacy of 5-FU [, ].
Q3: Can this compound itself be detected, and if so, how?
A: Yes, this compound can be directly detected and quantified using advanced analytical techniques like high-performance liquid chromatography (HPLC) and 19F nuclear magnetic resonance (NMR) spectroscopy [, , ]. These techniques have been instrumental in characterizing the metabolic profile of 5-FU in various biological samples, including plasma, urine, and tissue extracts.
Q4: How do researchers study the impact of this compound formation in preclinical models?
A: Researchers use in vivo models like mice bearing colon 38 tumors to investigate the effects of modulating this compound formation on 5-FU metabolism []. By administering compounds like EU before 5-FU, they can observe changes in the levels of this compound and other metabolites in different tissues using 19F NMR spectroscopy. These studies provide valuable insights into how altering 5-FU catabolism can impact its availability and potential antitumor activity.
Q5: What happens to this compound after it is formed?
A: this compound is further metabolized into alpha-fluoro-beta-alanine (FBAL) [, ]. FBAL is then excreted in the urine, representing a significant route of elimination for the breakdown products of 5-FU.
Q6: Does the formation of this compound vary across species?
A: Yes, the metabolic profile of 5-FU, including the formation and excretion of this compound, can vary significantly across species. For example, studies using 19F NMR spectroscopy have shown distinct differences in the urinary excretion patterns of FUPA and its metabolites between rats, mice, and humans [].
Q7: Are there other drugs or compounds that influence the metabolism of 5-FU and the formation of this compound ?
A: Yes, several compounds can influence 5-FU metabolism. For instance, using modulators like methotrexate, alpha-interferon, or high-dose leucovorin alongside 5-FU led to an increased ratio of anabolites to catabolites in murine colon carcinoma models []. This suggests a potential shift in 5-FU metabolism towards pathways favoring its antitumor activity. Conversely, N-(phosphonacetyl)-L-aspartate decreased the anabolite:catabolite ratio, potentially reducing 5-FU efficacy [].
Avertissement et informations sur les produits de recherche in vitro
Veuillez noter que tous les articles et informations sur les produits présentés sur BenchChem sont destinés uniquement à des fins informatives. Les produits disponibles à l'achat sur BenchChem sont spécifiquement conçus pour des études in vitro, qui sont réalisées en dehors des organismes vivants. Les études in vitro, dérivées du terme latin "in verre", impliquent des expériences réalisées dans des environnements de laboratoire contrôlés à l'aide de cellules ou de tissus. Il est important de noter que ces produits ne sont pas classés comme médicaments et n'ont pas reçu l'approbation de la FDA pour la prévention, le traitement ou la guérison de toute condition médicale, affection ou maladie. Nous devons souligner que toute forme d'introduction corporelle de ces produits chez les humains ou les animaux est strictement interdite par la loi. Il est essentiel de respecter ces directives pour assurer la conformité aux normes légales et éthiques en matière de recherche et d'expérimentation.