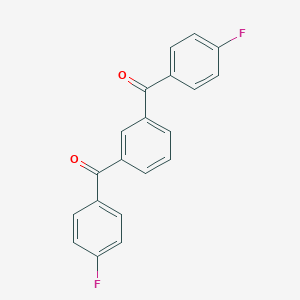
1,3-Bis(4-fluorobenzoyl)benzene
Vue d'ensemble
Description
1,3-Bis(4-fluorobenzoyl)benzene (CAS 108464-88-6) is an aromatic ketone with a central benzene ring substituted at the 1 and 3 positions by 4-fluorobenzoyl groups. Its molecular formula is C₂₀H₁₂F₂O₂ (molecular weight: 322.3 g/mol), and it exhibits a melting point of 181–183°C and a predicted boiling point of 476.3±40.0°C . The fluorine atoms enhance chemical stability and reduce polarity, making it valuable in polymer chemistry, particularly for synthesizing poly(aryl ether ketone)s (PAEKs) with tailored dielectric, thermal, and solubility properties . Applications span proton exchange membranes, high-performance plastics, and optical materials due to its conjugated π-system .
Méthodes De Préparation
Friedel-Crafts Acylation: Core Synthesis Mechanism
The primary route to 1,3-bis(4-fluorobenzoyl)benzene involves Friedel-Crafts acylation , leveraging isophthaloyl chloride and fluorobenzene in the presence of a Lewis acid catalyst. This method mirrors the synthesis of its structural isomer, 1,4-bis(4-fluorobenzoyl)benzene, but requires tailored adjustments to accommodate the meta-substitution pattern .
Reaction Conditions and Catalyst Optimization
The reaction proceeds via the following stoichiometry:
Key parameters include:
-
Catalyst : Aluminum chloride (AlCl₃) or aluminum bromide (AlBr₃) at 1.8–3.0 mol per mole of isophthaloyl chloride .
-
Temperature : Maintained below 70°C to suppress side reactions (e.g., halogen exchange or isomerization) .
-
Solvent : Excess fluorobenzene acts as both reactant and solvent, typically at 7–20 mol per mole of isophthaloyl chloride .
A comparative analysis of reaction conditions is provided in Table 1.
Table 1: Friedel-Crafts Acylation Conditions for this compound
Parameter | Optimal Range | Impact on Yield/Purity |
---|---|---|
Catalyst (AlCl₃) | 2.0–2.5 mol/mol | Maximizes electrophilic acylation |
Temperature | 35–65°C | Minimizes AlCl₃-induced fluorobenzene chlorination |
Fluorobenzene Excess | 10–16 mol/mol | Ensures complete diacylation |
Reaction Time | 3–6 hours | Balances completion vs. degradation |
Industrial-Scale Synthesis and Process Refinement
Industrial production prioritizes cost-effectiveness while maintaining >99.5% isomeric purity. Lessons from the synthesis of 1,4-bis(4-fluorobenzoyl)benzene inform best practices for the 1,3 isomer:
Catalyst Metering and Temperature Control
Gradual addition of AlCl₃ into a pre-mixed solution of isophthaloyl chloride and fluorobenzene prevents localized overheating, which promotes halogen exchange (e.g., F → Cl substitution). For example, metering 140 g of AlCl₃ over 1.5 hours at 25–30°C reduces o,p-isomer formation to <2% .
Work-Up and Purification
Post-reaction hydrolysis and phase separation are critical:
-
Hydrolysis : Quenching the reaction mixture with water deactivates AlCl₃ and precipitates the crude product.
-
Distillation : Excess fluorobenzene is recovered at 85°C (boiling point) for reuse .
-
Recrystallization : Chlorobenzene or toluene recrystallization elevates purity to >99.5%, as confirmed by HPLC .
Side Reactions and Byproduct Mitigation
Halogen Exchange and Isomerization
At temperatures >70°C, AlCl₃ catalyzes fluorobenzene chlorination, yielding 1-(4-chlorobenzoyl)-4-(4-fluorobenzoyl)benzene as a byproduct . This is minimized by:
-
Strict temperature control (<65°C).
-
Using fresh fluorobenzene to avoid Cl contamination.
Ortho-Substitution Byproducts
Meta-directing groups on isophthaloyl chloride favor para-substitution on fluorobenzene. However, ~1–2% o,p-isomers form due to steric effects, necessitating recrystallization .
Comparative Analysis with 1,4-Bis(4-fluorobenzoyl)benzene Synthesis
While both isomers share a synthetic backbone, key differences arise from their substitution patterns:
Table 2: 1,3 vs. 1,4 Isomer Synthesis Comparison
Analyse Des Réactions Chimiques
Types of Reactions
1,3-Bis(4-fluorobenzoyl)benzene undergoes various chemical reactions, including:
Substitution Reactions: The fluorine atoms can be substituted with other functional groups under appropriate conditions.
Polymerization: It can polymerize with compounds like bisphenol-A to form high molecular weight polymers
Common Reagents and Conditions
Substitution Reactions: Common reagents include nucleophiles such as amines or thiols. The reactions typically occur under mild conditions with the use of catalysts.
Polymerization: Solvents like N-methyl-2-pyrrolidinone and bases such as pyridine are commonly used
Major Products Formed
Substitution Reactions: Products include various substituted derivatives of this compound.
Polymerization: High molecular weight polymers, such as sulfonated poly(aryl ether ketone)s (SPAEK), are formed
Applications De Recherche Scientifique
Synthesis of 1,3-Bis(4-fluorobenzoyl)benzene
The synthesis of this compound typically involves the reaction of fluorobenzoyl chloride with benzene derivatives in the presence of Lewis acids such as aluminum chloride. The process can be optimized to yield high purity and significant quantities of the product, which is crucial for industrial applications .
Poly(aryl ether ketones) Synthesis
This compound serves as a key monomer in the synthesis of poly(aryl ether ketones), which are known for their excellent thermal stability, mechanical strength, and resistance to chemicals. These polymers find applications in:
- Aerospace components
- Electrical insulation
- Automotive parts
Proton Exchange Membrane Materials
Research has demonstrated that this compound can be used to develop sulfonated poly(aryl ether ketone)s (SPAEK), which are promising candidates for proton exchange membranes in fuel cells due to their high ionic conductivity and thermal stability .
High-Performance Plastics
The compound is also utilized as a precursor for high-performance plastics such as polyether ketosulfones and laminated materials. These materials are employed in environments requiring high strength and resistance to heat and chemicals .
Case Study 1: Fuel Cell Applications
In a study published in Journal of Membrane Science, researchers synthesized SPAEK from this compound, demonstrating its effectiveness as a proton exchange membrane with improved performance compared to traditional materials . The study highlighted the material's ability to maintain conductivity at elevated temperatures.
Case Study 2: Development of Polyimides
Another significant application involves the incorporation of this compound into polyimide formulations aimed at achieving low glass transition temperatures while maintaining thermal stability. This research indicated that the resulting polyimides exhibited enhanced mechanical properties suitable for aerospace applications .
Market Insights
The global market for this compound is projected to grow significantly due to its increasing use in high-performance materials. The compound's versatility in various industrial applications positions it as a valuable asset in material science research and development .
Mécanisme D'action
The mechanism of action of 1,3-Bis(4-fluorobenzoyl)benzene involves its ability to generate reactive oxygen species and activate nucleophilic protons on the benzene ring. This compound can bind to DNA via hydrogen bonding interactions with cytosine bases, leading to the inhibition of DNA synthesis. This mechanism is particularly relevant in its potential anticancer applications .
Comparaison Avec Des Composés Similaires
Comparison with Structurally Similar Compounds
1,4-Bis(4-fluorobenzoyl)benzene (BFBB)
- Structure : Substituted at the 1 and 4 positions of the central benzene ring.
- Properties :
- Melting Point : 218.5–219.5°C (higher than the 1,3-isomer due to symmetry) .
- Synthesis : Prepared via Friedel-Crafts acylation of terephthaloyl chloride with fluorobenzene using AlCl₃, yielding 88% purity in early methods .
- Applications : Used in PAEKs and polyether ketones. However, BFBB-based polymers exhibit higher dielectric constants (ε ≈ 3.0–3.2 at 1 MHz) compared to 1,3-isomer derivatives, limiting use in low-dielectric applications .
- Key Differences :
1,4-Di(4-fluorobenzoyl)cyclohexane (DFBCH)
- Structure: Cyclohexane ring replaces the central benzene, reducing polarity via non-coplanar geometry.
- Properties :
- Key Differences : Cyclohexane introduces conformational flexibility, enhancing solubility and reducing dielectric loss in high-frequency applications .
Sulfonated Derivatives
- 1,4-Bi(3-sodium sulfonate-4-fluorobenzoyl)benzene : Derived from BFBB via sulfonation, this compound forms proton-conductive membranes with ion exchange capacities (IEC) of 1.5–2.0 meq/g for fuel cells .
- Comparison : Sulfonated 1,3-isomers are less studied but may offer better solubility due to asymmetric substitution, though with trade-offs in mechanical strength .
Comparative Data Table
Research Findings and Implications
- 1,3-Isomer Advantages : Asymmetric substitution improves solubility and processability, enabling fabrication of flexible films for proton exchange membranes . Its dielectric properties (ε ≈ 3.0–3.2) are marginally better than BFBB but inferior to cyclohexane-based DFBCH .
- Thermal Performance: The 1,4-isomer’s higher melting point suits high-temperature applications, but its brittleness necessitates copolymerization with flexible monomers like DFBCH .
- Emerging Trends : Blending this compound-based PAEKs with π-electron-deficient polyimides enhances mechanical strength via supramolecular interactions, as shown in flexural strength studies (12–14 ksi) .
Activité Biologique
1,3-Bis(4-fluorobenzoyl)benzene is a compound that has garnered attention for its potential biological activities, particularly in the context of cancer research. This article synthesizes findings from various studies to provide a comprehensive overview of the biological activity of this compound, focusing on its mechanisms of action, efficacy in inhibiting cancer cell invasion, and its potential as a therapeutic agent.
Chemical Structure and Properties
This compound belongs to the class of benzoylbenzene derivatives, characterized by two fluorobenzoyl groups attached to a central benzene ring. Its molecular formula is CHFO, and it exhibits significant lipophilicity due to the presence of fluorine atoms, which can enhance its interaction with biological membranes.
Research has highlighted that this compound acts primarily as an inhibitor of cathepsin L, a cysteine protease implicated in tumor invasion and metastasis. The inhibition of cathepsin L can lead to decreased cancer cell migration and invasion, making it a promising candidate for anti-metastatic therapy.
Key Findings:
- Cathepsin L Inhibition : The compound demonstrated low IC50 values (14.4 nM), indicating potent inhibition against cathepsin L compared to other analogues .
- Selectivity : It exhibited selectivity for cathepsin L over cathepsin B, suggesting a targeted approach in cancer treatment .
In Vitro Studies
In vitro studies have shown that this compound effectively inhibits the invasive potential of various cancer cell lines:
- MDA-MB-231 Breast Cancer Cells : The thiosemicarbazone analogue derived from this compound inhibited invasion through Matrigel by 70% at a concentration of 10 μM .
- PC-3ML Prostate Cancer Cells : The compound significantly reduced the invasive capabilities by 92% at 5 μM .
In Vivo Studies
Initial in vivo assessments in mouse models have indicated that compounds related to this compound are well-tolerated and can delay tumor growth without significant cytotoxicity towards normal cells. This characteristic is crucial for developing effective anti-cancer therapies that minimize damage to healthy tissues.
Case Studies
Several studies have been conducted to evaluate the efficacy of this compound in different cancer models:
Study | Cancer Type | Treatment Concentration | Effectiveness |
---|---|---|---|
Breast | 10 μM | 70% invasion inhibition | |
Prostate | 5 μM | 92% invasion inhibition | |
Various | Not specified | Potent cathepsin L inhibition |
These findings support the potential use of this compound as part of a therapeutic strategy against metastatic cancers.
Q & A
Basic Research Questions
Q. What are the standard synthetic routes for preparing 1,3-Bis(4-fluorobenzoyl)benzene, and how is purity ensured?
this compound is synthesized via Friedel–Crafts acylation or nucleophilic aromatic substitution. A representative method involves sulfonation of the parent compound using 50% fuming sulfuric acid at 120°C for 12 hours, followed by neutralization with NaOH and NaCl for salt precipitation. Purification is achieved through multiple recrystallizations in ice water, yielding 50% pure product . Solvent selection (e.g., DMSO/甲苯 mixtures) and reflux conditions (3–20 hours) are critical for removing byproducts and unreacted reagents.
Q. Which analytical techniques are essential for confirming the structure and purity of this compound?
- FT-IR spectroscopy : Identifies carbonyl (C=O) stretches (~1650 cm⁻¹) and sulfonate groups (if modified) .
- NMR spectroscopy : ¹H NMR (400 MHz) resolves aromatic protons and fluorobenzoyl substituents, while ¹⁹F NMR confirms fluorine environments .
- Thermal analysis : Differential scanning calorimetry (DSC) and thermogravimetric analysis (TGA) assess thermal stability (e.g., decomposition above 300°C under nitrogen) .
- Elemental analysis : Validates C/H/F ratios to confirm stoichiometry.
Q. What are the key solubility and storage considerations for this compound?
The compound is sparingly soluble in polar aprotic solvents like DMSO but insoluble in water. Storage requires anhydrous conditions (desiccators) at 0–4°C to prevent hydrolysis of fluorobenzoyl groups. Use amber glass vials to avoid photodegradation .
Advanced Research Questions
Q. How is this compound functionalized for ion-exchange membrane applications?
Sulfonation with fuming sulfuric acid introduces sulfonate groups (-SO₃Na) at the 3-position of the fluorobenzoyl rings, forming 1,3-Bis(3-sodium sulfonate-4-fluorobenzoyl)benzene (BSFBZB). This derivative is copolymerized with 4,4’-dichlorodiphenyl sulfone and 4,4’-thiodibenzenethiol in DMSO/甲苯 under reflux, yielding sulfonated poly(arylene thioether) membranes. These membranes exhibit proton conductivity >0.1 S/cm at 80°C, suitable for fuel cells .
Q. What methodologies optimize copolymer synthesis for thermotropic liquid crystalline polymers?
Copolymers with 50–70 mol% biphenol are synthesized via nucleophilic substitution of this compound with 4,4’-biphenol and phenylhydroquinone. Reaction conditions (160°C, 24 hours in NMP) ensure high molecular weight (Mw >30 kDa). Liquid crystallinity is confirmed by polarized optical microscopy (schlieren textures) and DSC (glass transition at ~150°C, clearing points >280°C) .
Q. How do conflicting data on sulfonation efficiency arise, and how can they be resolved?
The 50% yield reported for BSFBZB synthesis may stem from competing side reactions (e.g., over-sulfonation or ring degradation). Optimization strategies include:
- Temperature control : Maintaining 120°C ± 2°C to balance reaction rate and selectivity.
- Stoichiometric adjustments : Using 1.2 equivalents of sulfonating agent to minimize byproducts.
- In-situ monitoring : Real-time FT-IR to track sulfonate group formation.
Q. What computational or experimental approaches elucidate electronic properties for optoelectronic applications?
Density functional theory (DFT) calculations predict HOMO/LUMO levels (~6.1 eV/2.4 eV) for fluorobenzoyl derivatives, aligning with experimental cyclic voltammetry data. Fluorescence studies (λem = 345–360 nm in THF) suggest potential as blue-emitting materials in OLEDs, though aggregation-caused quenching requires copolymer blending .
Q. Methodological Tables
Table 1: Key Synthetic Parameters for Sulfonated Derivatives
Parameter | Condition/Value | Reference |
---|---|---|
Sulfonation temperature | 120°C | |
Reaction time | 12 hours | |
Neutralization agent | NaOH/NaCl | |
Recrystallization steps | 5× in H₂O | |
Final yield | 50% |
Table 2: Thermal Properties of Copolymers
Copolymer Composition | Tg (°C) | Td (°C) | Application | Reference |
---|---|---|---|---|
50 mol% biphenol | 150 | 320 | Liquid crystals | |
Sulfonated derivative | 180 | 350 | Ion-exchange membranes |
Propriétés
IUPAC Name |
[3-(4-fluorobenzoyl)phenyl]-(4-fluorophenyl)methanone | |
---|---|---|
Source | PubChem | |
URL | https://pubchem.ncbi.nlm.nih.gov | |
Description | Data deposited in or computed by PubChem | |
InChI |
InChI=1S/C20H12F2O2/c21-17-8-4-13(5-9-17)19(23)15-2-1-3-16(12-15)20(24)14-6-10-18(22)11-7-14/h1-12H | |
Source | PubChem | |
URL | https://pubchem.ncbi.nlm.nih.gov | |
Description | Data deposited in or computed by PubChem | |
InChI Key |
PISLKPDKKIDMQT-UHFFFAOYSA-N | |
Source | PubChem | |
URL | https://pubchem.ncbi.nlm.nih.gov | |
Description | Data deposited in or computed by PubChem | |
Canonical SMILES |
C1=CC(=CC(=C1)C(=O)C2=CC=C(C=C2)F)C(=O)C3=CC=C(C=C3)F | |
Source | PubChem | |
URL | https://pubchem.ncbi.nlm.nih.gov | |
Description | Data deposited in or computed by PubChem | |
Molecular Formula |
C20H12F2O2 | |
Source | PubChem | |
URL | https://pubchem.ncbi.nlm.nih.gov | |
Description | Data deposited in or computed by PubChem | |
DSSTOX Substance ID |
DTXSID20350852 | |
Record name | 1,3-Bis(4-fluorobenzoyl)benzene | |
Source | EPA DSSTox | |
URL | https://comptox.epa.gov/dashboard/DTXSID20350852 | |
Description | DSSTox provides a high quality public chemistry resource for supporting improved predictive toxicology. | |
Molecular Weight |
322.3 g/mol | |
Source | PubChem | |
URL | https://pubchem.ncbi.nlm.nih.gov | |
Description | Data deposited in or computed by PubChem | |
CAS No. |
108464-88-6 | |
Record name | 1,3-Bis(4-fluorobenzoyl)benzene | |
Source | EPA DSSTox | |
URL | https://comptox.epa.gov/dashboard/DTXSID20350852 | |
Description | DSSTox provides a high quality public chemistry resource for supporting improved predictive toxicology. | |
Synthesis routes and methods I
Procedure details
Synthesis routes and methods II
Procedure details
Retrosynthesis Analysis
AI-Powered Synthesis Planning: Our tool employs the Template_relevance Pistachio, Template_relevance Bkms_metabolic, Template_relevance Pistachio_ringbreaker, Template_relevance Reaxys, Template_relevance Reaxys_biocatalysis model, leveraging a vast database of chemical reactions to predict feasible synthetic routes.
One-Step Synthesis Focus: Specifically designed for one-step synthesis, it provides concise and direct routes for your target compounds, streamlining the synthesis process.
Accurate Predictions: Utilizing the extensive PISTACHIO, BKMS_METABOLIC, PISTACHIO_RINGBREAKER, REAXYS, REAXYS_BIOCATALYSIS database, our tool offers high-accuracy predictions, reflecting the latest in chemical research and data.
Strategy Settings
Precursor scoring | Relevance Heuristic |
---|---|
Min. plausibility | 0.01 |
Model | Template_relevance |
Template Set | Pistachio/Bkms_metabolic/Pistachio_ringbreaker/Reaxys/Reaxys_biocatalysis |
Top-N result to add to graph | 6 |
Feasible Synthetic Routes
Avertissement et informations sur les produits de recherche in vitro
Veuillez noter que tous les articles et informations sur les produits présentés sur BenchChem sont destinés uniquement à des fins informatives. Les produits disponibles à l'achat sur BenchChem sont spécifiquement conçus pour des études in vitro, qui sont réalisées en dehors des organismes vivants. Les études in vitro, dérivées du terme latin "in verre", impliquent des expériences réalisées dans des environnements de laboratoire contrôlés à l'aide de cellules ou de tissus. Il est important de noter que ces produits ne sont pas classés comme médicaments et n'ont pas reçu l'approbation de la FDA pour la prévention, le traitement ou la guérison de toute condition médicale, affection ou maladie. Nous devons souligner que toute forme d'introduction corporelle de ces produits chez les humains ou les animaux est strictement interdite par la loi. Il est essentiel de respecter ces directives pour assurer la conformité aux normes légales et éthiques en matière de recherche et d'expérimentation.