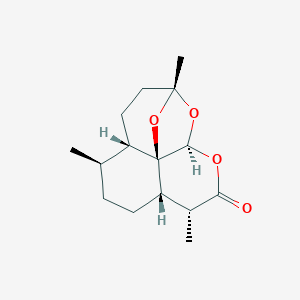
Deoxyartemisinin
Vue d'ensemble
Description
Deoxyartemisinin is a natural product found in Artemisia annua, Artemisia carvifolia, and Artemisia apiacea . It lacks the peroxide bridge, which makes it biologically inert .
Synthesis Analysis
Deoxyartemisinin and 9-ene-10-deoxyartemisinin were synthesized after simple handling with boron trifluoride/diethyl ether and sodium borohydride . The structural changes of deoxyartemisinin obviously affect their physical and chemical properties, resulting in different pharmacokinetic behaviors and pharmacological activities in vivo .
Molecular Structure Analysis
The molecular formula of Deoxyartemisinin is C15H22O4 . The InChI is InChI=1S/C15H22O4/c1-8-4-5-11-9 (2)12 (16)17-13-15 (11)10 (8)6-7-14 (3,18-13)19-15/h8-11,13H,4-7H2,1-3H3/t8-,9-,10+,11+,13-,14-,15-/m1/s1 . The Canonical SMILES is CC1CCC2C (C (=O)OC3C24C1CCC (O3) (O4)C) .
Chemical Reactions Analysis
The absorption, distribution, metabolism, excretion (ADME) properties of artemisinin, deoxyartemisinin, and 10-deoxoartemisinin were first predicted using QikProp software . The oral bioavailability of artemisinin was calculated to be 12.2 ± 0.832%, which was about 7 times higher than that of deoxyartemisinin (1.60 ± 0.317%) .
Physical And Chemical Properties Analysis
The molecular weight of Deoxyartemisinin is 266.33 g/mol . The structural changes of deoxyartemisinin and 10-deoxoartemisinin obviously affect their physical and chemical properties, resulting in different pharmacokinetic behaviors and pharmacological activities in vivo .
Applications De Recherche Scientifique
Malaria Treatment
Deoxyartemisinin plays a crucial role in the treatment of malaria. Artemisinin-based combination therapy (ACT) is the preferred choice for malaria treatment recommended by the World Health Organization . The compound’s ability to combat malaria parasites makes it an essential component in the global fight against this life-threatening disease.
Antimicrobial Activity
Research has shown that Deoxyartemisinin exhibits antibacterial activity against various strains of bacteria. It has been reported to show activity against Staphylococcus aureus, S. epidermidis, and S. mutans at a minimum inhibitory concentration (MIC) of 1 mg/mL, which is more effective than Artemisinin, whose MIC is greater than 2 mg/mL . This suggests its potential use as an antimicrobial agent in medical treatments.
Biosynthesis Advancements
The biosynthesis of Artemisinin and its derivatives, including Deoxyartemisinin, has seen significant advancements. The application of synthetic biology to produce Artemisinin in vivo using microorganisms such as Escherichia coli and Saccharomyces cerevisiae has shown promising results. This approach may lead to more efficient production methods for Deoxyartemisinin .
Genetic Engineering in Plant Production
Genetic engineering techniques have been applied to the plant Artemisia annua, the natural source of Artemisinin, to increase its yield. This includes overexpressing genes related to the Artemisinin biosynthesis pathway, blocking competitive pathways, and regulating transcription factors. These methods could potentially enhance the production of Deoxyartemisinin .
Biotransformation Through Fungal Strains
Deoxyartemisinin can be obtained through the biotransformation of Artemisinin mediated by fungal strains. For instance, the fungus Aspergillus flavus has been capable of transforming Artemisinin to Deoxyartemisinin in higher yields than previous reports . This process could be a viable method for producing Deoxyartemisinin on a larger scale.
Pharmaceutical Applications
Deoxyartemisinin’s pharmaceutical applications are vast due to its bioactive properties. Its rapid metabolism in the body makes it a compound of interest for developing new drugs and treatment methods. The metabolites of Artemisinin, including Deoxyartemisinin, are studied for their potential uses in various pharmaceutical applications .
Mécanisme D'action
Target of Action
Deoxyartemisinin, a derivative of artemisinin, primarily targets the mitochondria of malarial parasites . The mitochondria play a crucial role in executing the action of artemisinin and its derivatives .
Mode of Action
Deoxyartemisinin interacts with its target, the malarial mitochondria, by inducing a rapid and dramatic production of reactive oxygen species (ROS) . Unlike artemisinin and its other derivatives, deoxyartemisinin lacks an endoperoxide bridge, which plays a key role in their inhibitory action .
Biochemical Pathways
Deoxyartemisinin is part of the artemisinin biosynthetic pathway, which is initiated by the enzyme Amorpha-4,11-diene synthase (ADS) . This pathway is crucial for the emergence of the specialized artemisinin biosynthetic pathway in the species Artemisia annua .
Pharmacokinetics
The pharmacokinetics of deoxyartemisinin involve its absorption, distribution, metabolism, and excretion (ADME) properties . The oral bioavailability of deoxyartemisinin is calculated to be 1.60 ± 0.317%, which is significantly lower than that of artemisinin (12.2 ± 0.832%) . This suggests that the absence of the peroxide bridge in deoxyartemisinin might decrease its absorption rate in the gastrointestinal tract .
Result of Action
The primary result of deoxyartemisinin’s action is the inhibition of malarial growth . This differentiates it from other artemisinin derivatives, which induce ROS production and depolarization in malarial mitochondria .
Action Environment
The action of deoxyartemisinin, like other artemisinin derivatives, can be influenced by environmental factors. For instance, the addition of an iron chelator can drastically reduce the activity of the mitochondrial electron transport chain (ETC) and mitigate the ROS production induced by artemisinin .
Safety and Hazards
Propriétés
IUPAC Name |
(1S,4S,5R,8S,9R,12S,13R)-1,5,9-trimethyl-11,14,15-trioxatetracyclo[10.2.1.04,13.08,13]pentadecan-10-one | |
---|---|---|
Source | PubChem | |
URL | https://pubchem.ncbi.nlm.nih.gov | |
Description | Data deposited in or computed by PubChem | |
InChI |
InChI=1S/C15H22O4/c1-8-4-5-11-9(2)12(16)17-13-15(11)10(8)6-7-14(3,18-13)19-15/h8-11,13H,4-7H2,1-3H3/t8-,9-,10+,11+,13-,14-,15-/m1/s1 | |
Source | PubChem | |
URL | https://pubchem.ncbi.nlm.nih.gov | |
Description | Data deposited in or computed by PubChem | |
InChI Key |
ZQGMLVQZBIKKMP-NNWCWBAJSA-N | |
Source | PubChem | |
URL | https://pubchem.ncbi.nlm.nih.gov | |
Description | Data deposited in or computed by PubChem | |
Canonical SMILES |
CC1CCC2C(C(=O)OC3C24C1CCC(O3)(O4)C)C | |
Source | PubChem | |
URL | https://pubchem.ncbi.nlm.nih.gov | |
Description | Data deposited in or computed by PubChem | |
Isomeric SMILES |
C[C@@H]1CC[C@H]2[C@H](C(=O)O[C@H]3[C@@]24[C@H]1CC[C@](O3)(O4)C)C | |
Source | PubChem | |
URL | https://pubchem.ncbi.nlm.nih.gov | |
Description | Data deposited in or computed by PubChem | |
Molecular Formula |
C15H22O4 | |
Source | PubChem | |
URL | https://pubchem.ncbi.nlm.nih.gov | |
Description | Data deposited in or computed by PubChem | |
Molecular Weight |
266.33 g/mol | |
Source | PubChem | |
URL | https://pubchem.ncbi.nlm.nih.gov | |
Description | Data deposited in or computed by PubChem | |
Product Name |
Deoxyartemisinin | |
CAS RN |
72826-63-2 | |
Record name | Deoxyartemisinin | |
Source | CAS Common Chemistry | |
URL | https://commonchemistry.cas.org/detail?cas_rn=72826-63-2 | |
Description | CAS Common Chemistry is an open community resource for accessing chemical information. Nearly 500,000 chemical substances from CAS REGISTRY cover areas of community interest, including common and frequently regulated chemicals, and those relevant to high school and undergraduate chemistry classes. This chemical information, curated by our expert scientists, is provided in alignment with our mission as a division of the American Chemical Society. | |
Explanation | The data from CAS Common Chemistry is provided under a CC-BY-NC 4.0 license, unless otherwise stated. | |
Record name | Deoxyartemisinin | |
Source | ChemIDplus | |
URL | https://pubchem.ncbi.nlm.nih.gov/substance/?source=chemidplus&sourceid=0072826632 | |
Description | ChemIDplus is a free, web search system that provides access to the structure and nomenclature authority files used for the identification of chemical substances cited in National Library of Medicine (NLM) databases, including the TOXNET system. | |
Record name | 2-DEOXYARTEMISININ | |
Source | FDA Global Substance Registration System (GSRS) | |
URL | https://gsrs.ncats.nih.gov/ginas/app/beta/substances/J59T2407WZ | |
Description | The FDA Global Substance Registration System (GSRS) enables the efficient and accurate exchange of information on what substances are in regulated products. Instead of relying on names, which vary across regulatory domains, countries, and regions, the GSRS knowledge base makes it possible for substances to be defined by standardized, scientific descriptions. | |
Explanation | Unless otherwise noted, the contents of the FDA website (www.fda.gov), both text and graphics, are not copyrighted. They are in the public domain and may be republished, reprinted and otherwise used freely by anyone without the need to obtain permission from FDA. Credit to the U.S. Food and Drug Administration as the source is appreciated but not required. | |
Retrosynthesis Analysis
AI-Powered Synthesis Planning: Our tool employs the Template_relevance Pistachio, Template_relevance Bkms_metabolic, Template_relevance Pistachio_ringbreaker, Template_relevance Reaxys, Template_relevance Reaxys_biocatalysis model, leveraging a vast database of chemical reactions to predict feasible synthetic routes.
One-Step Synthesis Focus: Specifically designed for one-step synthesis, it provides concise and direct routes for your target compounds, streamlining the synthesis process.
Accurate Predictions: Utilizing the extensive PISTACHIO, BKMS_METABOLIC, PISTACHIO_RINGBREAKER, REAXYS, REAXYS_BIOCATALYSIS database, our tool offers high-accuracy predictions, reflecting the latest in chemical research and data.
Strategy Settings
Precursor scoring | Relevance Heuristic |
---|---|
Min. plausibility | 0.01 |
Model | Template_relevance |
Template Set | Pistachio/Bkms_metabolic/Pistachio_ringbreaker/Reaxys/Reaxys_biocatalysis |
Top-N result to add to graph | 6 |
Feasible Synthetic Routes
Q & A
A: Deoxyartemisinin lacks the endoperoxide bridge present in Artemisinin. [, , , ] This structural difference significantly impacts its antimalarial activity, making Deoxyartemisinin less potent than Artemisinin. [, , , ] While the peroxide bridge is considered crucial for Artemisinin's antimalarial action, Deoxyartemisinin may exert its effects through alternative mechanisms, warranting further investigation. []
A: Research suggests that mitochondria are a direct target of Artemisinin and its derivatives. [] These compounds, particularly those with the endoperoxide bridge, induce rapid reactive oxygen species (ROS) production in isolated yeast and malarial mitochondria but not in mammalian mitochondria, leading to mitochondrial dysfunction. [] This selective toxicity towards malarial mitochondria underscores the importance of this organelle in the antimalarial action of these compounds.
A: The molecular formula of Deoxyartemisinin is C15H24O4, and its molecular weight is 268.35 g/mol. []
A: Researchers employ a range of spectroscopic techniques to elucidate the structure of Deoxyartemisinin, including Infrared Spectroscopy (IR), Proton and Carbon-13 Nuclear Magnetic Resonance (1H and 13C NMR), Heteronuclear Single Quantum Coherence (HSQC), Heteronuclear Multiple Bond Correlation (HMBC), Correlation Spectroscopy (COSY), Nuclear Overhauser Effect Spectroscopy (NOESY), and High-Resolution Electrospray Ionization Mass Spectrometry (HR-ESIMS). [, , ]
A: Yes, several fungal strains, including Aspergillus flavus, Aspergillus niger, Cunninghamella elegans, and Mucor polymorphosporus, have been identified to biotransform Artemisinin into Deoxyartemisinin. [, , , , , , ] This microbial transformation offers a potentially sustainable and efficient approach for Deoxyartemisinin production.
A: Yes, computational techniques, including molecular docking and Comparative Molecular Field Analysis (CoMFA), have been employed to investigate Deoxyartemisinin and its derivatives. [, , , ] These studies provide insights into their potential interactions with target proteins and guide the design of novel analogues with improved activity.
A: Studies exploring the structure-activity relationship (SAR) of Deoxyartemisinin and its derivatives reveal that modifications at the C-9 position can significantly influence their antimalarial and antileishmanial properties. [, ] Specifically, substitutions at the C-9β position generally enhance activity compared to C-9α substitutions, suggesting the importance of spatial orientation for optimal target interactions.
A: Formulating Deoxyartemisinin presents challenges related to its inherent physicochemical properties. [, ] Strategies to enhance its stability, solubility, and bioavailability are crucial for developing effective pharmaceutical formulations. [, ] These strategies may include using different drug delivery systems, such as nanoparticles or liposomes, to protect the compound from degradation and improve its pharmacokinetic profile.
A: The oral bioavailability of Deoxyartemisinin (1.60 ± 0.317%) is significantly lower than that of Artemisinin (12.2 ± 0.832%). [] This difference highlights the impact of the endoperoxide bridge on absorption and underscores the need for alternative delivery strategies or structural modifications to improve the bioavailability of Deoxyartemisinin.
A: Both Artemisinin and Deoxyartemisinin undergo extensive metabolism, primarily in the liver. [, , , ] Common metabolic transformations include hydroxylation, deoxygenation, and glucuronidation. [, ] Understanding these metabolic pathways is crucial for optimizing drug efficacy and minimizing potential drug-drug interactions.
A: Deoxyartemisinin's activity has been evaluated against various targets using in vitro models. These include assays against Plasmodium falciparum to assess its antimalarial potential, as well as evaluations against tumor cell lines like EN2, A549, HCT116, and MCF7 to investigate its cytotoxic effects. [, , ] These in vitro studies provide valuable insights into the compound's biological activity and guide further research in specific therapeutic areas.
A: Studies in rats have shown that administering Artemisinin as part of dried leaves of Artemisia annua (DLA) significantly enhances its bioavailability compared to delivering pure Artemisinin. [] This suggests that other phytochemicals present in DLA may inhibit the first-pass metabolism of Artemisinin, leading to higher concentrations of the active compound reaching the systemic circulation.
A: Researchers commonly utilize High-Performance Liquid Chromatography (HPLC) coupled with various detection methods, such as fluorescence detection or mass spectrometry (MS), to quantify Deoxyartemisinin and its metabolites in various matrices. [, , , , ] Gas Chromatography-Mass Spectrometry (GC-MS) is also employed for the identification and quantification of these compounds in biological samples. [, , , ]
A: While Deoxyartemisinin demonstrates lower antimalarial potency compared to Artemisinin, it may present advantages in terms of stability and potentially lower production costs. [, , , ] Further research is necessary to fully explore its therapeutic potential and evaluate its suitability as an alternative to Artemisinin, considering factors like efficacy, safety, and cost-effectiveness.
Avertissement et informations sur les produits de recherche in vitro
Veuillez noter que tous les articles et informations sur les produits présentés sur BenchChem sont destinés uniquement à des fins informatives. Les produits disponibles à l'achat sur BenchChem sont spécifiquement conçus pour des études in vitro, qui sont réalisées en dehors des organismes vivants. Les études in vitro, dérivées du terme latin "in verre", impliquent des expériences réalisées dans des environnements de laboratoire contrôlés à l'aide de cellules ou de tissus. Il est important de noter que ces produits ne sont pas classés comme médicaments et n'ont pas reçu l'approbation de la FDA pour la prévention, le traitement ou la guérison de toute condition médicale, affection ou maladie. Nous devons souligner que toute forme d'introduction corporelle de ces produits chez les humains ou les animaux est strictement interdite par la loi. Il est essentiel de respecter ces directives pour assurer la conformité aux normes légales et éthiques en matière de recherche et d'expérimentation.