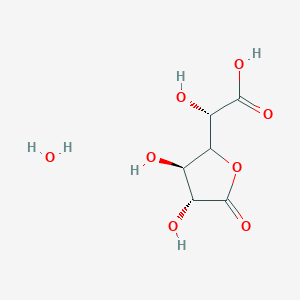
D-Acide saccharique 1,4-lactone monohydraté
Vue d'ensemble
Description
La D-Acide saccharique 1,4-lactone (hydrate) est un puissant inhibiteur de l'enzyme β-glucuronidase. Elle est largement utilisée dans la recherche scientifique en raison de sa capacité à inhiber la β-glucuronidase, une enzyme impliquée dans le métabolisme des glucides complexes .
Applications De Recherche Scientifique
D-Saccharic Acid 1,4-lactone (hydrate) has a wide range of applications in scientific research:
Mécanisme D'action
Target of Action
The primary target of D-Saccharic acid 1,4-lactone monohydrate (DSAL) is the β-glucuronidase enzyme . This enzyme plays a crucial role in the metabolism of glucuronides, which are key components in the detoxification of xenobiotics and endobiotics.
Mode of Action
DSAL acts as a potent inhibitor of the β-glucuronidase enzyme . By inhibiting this enzyme, DSAL prevents the breakdown of glucuronides, thereby influencing the metabolism of various substances within the body. Additionally, DSAL potentially inhibits Klotho deglycosylation of excitatory amino acid transporters (EAATs) and peptide transporters (PEPTs) .
Pharmacokinetics
Given its solubility in water , it can be inferred that DSAL may have good bioavailability
Result of Action
The inhibition of β-glucuronidase by DSAL can lead to an increase in the levels of glucuronides in the body. This can potentially influence the detoxification processes and the metabolism of various substances. DSAL’s action has been associated with anticarcinogenic, detoxifying, and antioxidant properties .
Action Environment
The action, efficacy, and stability of DSAL can be influenced by various environmental factors. For instance, the pH of the environment can affect the ionization state of DSAL, potentially influencing its interaction with the β-glucuronidase enzyme. The temperature can also affect the stability of DSAL, as it is recommended to be stored at 2-8°C .
Analyse Biochimique
Biochemical Properties
D-Saccharic acid 1,4-lactone monohydrate has the ability to inhibit the activity of β-glucuronidase enzyme . This interaction with β-glucuronidase enzyme plays a significant role in its biochemical reactions. It potentially inhibits Klotho deglycosylation of excitatory amino acid transporters (EAATs) and peptide transporters (PEPTs) .
Cellular Effects
The effects of D-Saccharic acid 1,4-lactone monohydrate on various types of cells and cellular processes are significant. It influences cell function by inhibiting β-glucuronidase enzyme, which plays a crucial role in cellular metabolism .
Molecular Mechanism
At the molecular level, D-Saccharic acid 1,4-lactone monohydrate exerts its effects through binding interactions with biomolecules, specifically the β-glucuronidase enzyme . By inhibiting this enzyme, it can cause changes in gene expression and cellular metabolism .
Temporal Effects in Laboratory Settings
It is known that it has a significant inhibitory effect on the β-glucuronidase enzyme .
Metabolic Pathways
D-Saccharic acid 1,4-lactone monohydrate is involved in the metabolic pathway of the β-glucuronidase enzyme . It interacts with this enzyme, potentially affecting metabolic flux or metabolite levels .
Méthodes De Préparation
Voies de synthèse et conditions de réaction
La D-Acide saccharique 1,4-lactone (hydrate) est généralement synthétisée par oxydation du D-glucose ou de l'acide D-glucuronique. Le processus implique l'utilisation d'oxydants forts tels que l'acide nitrique ou le permanganate de potassium dans des conditions contrôlées . La réaction est suivie d'une lactonisation pour former la structure cyclique de la lactone.
Méthodes de production industrielle
La production industrielle de D-Acide saccharique 1,4-lactone (hydrate) implique souvent une fermentation microbienne. Des micro-organismes spécifiques, tels que certaines souches de champignons ou de levures, sont utilisés pour faire fermenter le glucose, produisant de l'acide glucarique. Celui-ci est ensuite traité avec une base pour former la lactone, qui est ensuite cristallisée à partir de la solution .
Analyse Des Réactions Chimiques
Types de réactions
La D-Acide saccharique 1,4-lactone (hydrate) subit diverses réactions chimiques, notamment :
Oxydation : Elle peut être oxydée davantage pour produire différents dérivés.
Réduction : Le cycle lactone peut être réduit pour former le diol correspondant.
Substitution : Elle peut participer à des réactions de substitution où des groupes fonctionnels sont remplacés.
Réactifs et conditions communs
Oxydation : Les oxydants courants comprennent l'acide nitrique et le permanganate de potassium.
Réduction : Des agents réducteurs tels que le borohydrure de sodium ou l'hydrure de lithium et d'aluminium sont utilisés.
Substitution : Divers nucléophiles peuvent être utilisés en conditions acides ou basiques.
Principaux produits
Les principaux produits formés à partir de ces réactions comprennent différents dérivés de l'acide glucarique, des diols et des lactones substituées .
Applications de la recherche scientifique
La D-Acide saccharique 1,4-lactone (hydrate) a une large gamme d'applications dans la recherche scientifique :
Biologie : Elle est utilisée dans des études impliquant l'inhibition enzymatique, en particulier la β-glucuronidase.
Industrie : Elle est utilisée dans la production de divers produits pharmaceutiques et comme réactif biochimique.
Mécanisme d'action
La D-Acide saccharique 1,4-lactone (hydrate) exerce ses effets principalement par l'inhibition de la β-glucuronidase. Cette enzyme est responsable de l'hydrolyse des glucuronides, qui sont impliqués dans la détoxification de diverses substances dans l'organisme. En inhibant la β-glucuronidase, la D-Acide saccharique 1,4-lactone (hydrate) empêche la dégradation des glucuronides, améliorant ainsi le processus de détoxification . De plus, elle peut inhiber la déglycosylation des transporteurs d'acides aminés excitatoires et des transporteurs de peptides .
Comparaison Avec Des Composés Similaires
Composés similaires
D-Glucaro-1,4-lactone : Autre nom de la D-Acide saccharique 1,4-lactone (hydrate).
D-Saccharolactone : Un composé similaire avec des propriétés inhibitrices comparables.
Unicité
La D-Acide saccharique 1,4-lactone (hydrate) est unique en raison de sa puissante inhibition de la β-glucuronidase et de sa large gamme d'activités biologiques. Sa capacité à agir comme agent standard dans le développement de nouveaux inhibiteurs la distingue des autres composés similaires .
Propriétés
IUPAC Name |
(2S)-2-[(2S,3R,4R)-3,4-dihydroxy-5-oxooxolan-2-yl]-2-hydroxyacetic acid;hydrate | |
---|---|---|
Source | PubChem | |
URL | https://pubchem.ncbi.nlm.nih.gov | |
Description | Data deposited in or computed by PubChem | |
InChI |
InChI=1S/C6H8O7.H2O/c7-1-2(8)6(12)13-4(1)3(9)5(10)11;/h1-4,7-9H,(H,10,11);1H2/t1-,2-,3+,4+;/m1./s1 | |
Source | PubChem | |
URL | https://pubchem.ncbi.nlm.nih.gov | |
Description | Data deposited in or computed by PubChem | |
InChI Key |
NPFKVZHSFSFLPU-QGBSHYGGSA-N | |
Source | PubChem | |
URL | https://pubchem.ncbi.nlm.nih.gov | |
Description | Data deposited in or computed by PubChem | |
Canonical SMILES |
C1(C(C(=O)OC1C(C(=O)O)O)O)O.O | |
Source | PubChem | |
URL | https://pubchem.ncbi.nlm.nih.gov | |
Description | Data deposited in or computed by PubChem | |
Isomeric SMILES |
[C@H]1([C@H](C(=O)O[C@@H]1[C@@H](C(=O)O)O)O)O.O | |
Source | PubChem | |
URL | https://pubchem.ncbi.nlm.nih.gov | |
Description | Data deposited in or computed by PubChem | |
Molecular Formula |
C6H10O8 | |
Source | PubChem | |
URL | https://pubchem.ncbi.nlm.nih.gov | |
Description | Data deposited in or computed by PubChem | |
Molecular Weight |
210.14 g/mol | |
Source | PubChem | |
URL | https://pubchem.ncbi.nlm.nih.gov | |
Description | Data deposited in or computed by PubChem | |
Retrosynthesis Analysis
AI-Powered Synthesis Planning: Our tool employs the Template_relevance Pistachio, Template_relevance Bkms_metabolic, Template_relevance Pistachio_ringbreaker, Template_relevance Reaxys, Template_relevance Reaxys_biocatalysis model, leveraging a vast database of chemical reactions to predict feasible synthetic routes.
One-Step Synthesis Focus: Specifically designed for one-step synthesis, it provides concise and direct routes for your target compounds, streamlining the synthesis process.
Accurate Predictions: Utilizing the extensive PISTACHIO, BKMS_METABOLIC, PISTACHIO_RINGBREAKER, REAXYS, REAXYS_BIOCATALYSIS database, our tool offers high-accuracy predictions, reflecting the latest in chemical research and data.
Strategy Settings
Precursor scoring | Relevance Heuristic |
---|---|
Min. plausibility | 0.01 |
Model | Template_relevance |
Template Set | Pistachio/Bkms_metabolic/Pistachio_ringbreaker/Reaxys/Reaxys_biocatalysis |
Top-N result to add to graph | 6 |
Feasible Synthetic Routes
Avertissement et informations sur les produits de recherche in vitro
Veuillez noter que tous les articles et informations sur les produits présentés sur BenchChem sont destinés uniquement à des fins informatives. Les produits disponibles à l'achat sur BenchChem sont spécifiquement conçus pour des études in vitro, qui sont réalisées en dehors des organismes vivants. Les études in vitro, dérivées du terme latin "in verre", impliquent des expériences réalisées dans des environnements de laboratoire contrôlés à l'aide de cellules ou de tissus. Il est important de noter que ces produits ne sont pas classés comme médicaments et n'ont pas reçu l'approbation de la FDA pour la prévention, le traitement ou la guérison de toute condition médicale, affection ou maladie. Nous devons souligner que toute forme d'introduction corporelle de ces produits chez les humains ou les animaux est strictement interdite par la loi. Il est essentiel de respecter ces directives pour assurer la conformité aux normes légales et éthiques en matière de recherche et d'expérimentation.