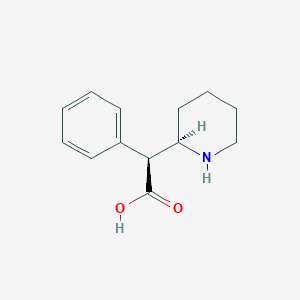
D-erythro-Ritalinic Acid
Vue d'ensemble
Description
D-erythro-Ritalinic Acid, also known as Ritalinic acid, is a substituted phenethylamine and an inactive major metabolite of the psychostimulant drugs methylphenidate, dexmethylphenidate, and ethylphenidate . When administered orally, methylphenidate is extensively metabolized in the liver by hydrolysis of the ester group yielding ritalinic acid .
Synthesis Analysis
D-erythro-Ritalinic Acid (dRA) can be synthesized from dl-Ritalinic acid (dlRA). The d- and l- isomers are separated by resolution using a resolving agent, (+)-dibenzoyl-D-tartaric acid .Molecular Structure Analysis
The structure of dRA is characterized by spectroscopic techniques such as Infrared spectroscopy (IR), mass spectrometry, and Nuclear Magnetic Resonance (NMR) .Chemical Reactions Analysis
D-erythro-Ritalinic Acid is a major metabolite of methylphenidate. It is formed through the hydrolysis of methylphenidate, a process catalyzed by carboxylesterase 1 (CES1A1) . CES1A1 exhibits six times higher enantioselectivity, preferring l-threo-enantiomer over d-threo-enantiomer .Physical And Chemical Properties Analysis
The physical and chemical properties of D-erythro-Ritalinic Acid can be analyzed using various techniques such as Liquid Chromatography-Mass Spectrometry (LC-MS/MS) .Safety and Hazards
D-erythro-Ritalinic Acid is considered hazardous by the 2012 OSHA Hazard Communication Standard . It is harmful if swallowed and causes serious eye irritation . Safety measures include washing hands and face thoroughly after handling, not eating, drinking, or smoking when using this product, and wearing protective gloves/clothing/eye protection/face protection .
Orientations Futures
Understanding the metabolomics of methylphenidate, including the role of D-erythro-Ritalinic Acid, can provide further insights regarding individual contribution for methylphenidate’s pharmacodynamics and toxicological effects, especially if ethanol is co-consumed . This knowledge is needed for a more efficacious therapeutic drug monitoring .
Propriétés
IUPAC Name |
(2S)-2-phenyl-2-[(2R)-piperidin-2-yl]acetic acid | |
---|---|---|
Source | PubChem | |
URL | https://pubchem.ncbi.nlm.nih.gov | |
Description | Data deposited in or computed by PubChem | |
InChI |
InChI=1S/C13H17NO2/c15-13(16)12(10-6-2-1-3-7-10)11-8-4-5-9-14-11/h1-3,6-7,11-12,14H,4-5,8-9H2,(H,15,16)/t11-,12+/m1/s1 | |
Source | PubChem | |
URL | https://pubchem.ncbi.nlm.nih.gov | |
Description | Data deposited in or computed by PubChem | |
InChI Key |
INGSNVSERUZOAK-NEPJUHHUSA-N | |
Source | PubChem | |
URL | https://pubchem.ncbi.nlm.nih.gov | |
Description | Data deposited in or computed by PubChem | |
Canonical SMILES |
C1CCNC(C1)C(C2=CC=CC=C2)C(=O)O | |
Source | PubChem | |
URL | https://pubchem.ncbi.nlm.nih.gov | |
Description | Data deposited in or computed by PubChem | |
Isomeric SMILES |
C1CCN[C@H](C1)[C@H](C2=CC=CC=C2)C(=O)O | |
Source | PubChem | |
URL | https://pubchem.ncbi.nlm.nih.gov | |
Description | Data deposited in or computed by PubChem | |
Molecular Formula |
C13H17NO2 | |
Source | PubChem | |
URL | https://pubchem.ncbi.nlm.nih.gov | |
Description | Data deposited in or computed by PubChem | |
Molecular Weight |
219.28 g/mol | |
Source | PubChem | |
URL | https://pubchem.ncbi.nlm.nih.gov | |
Description | Data deposited in or computed by PubChem | |
Product Name |
D-erythro-Ritalinic Acid |
Retrosynthesis Analysis
AI-Powered Synthesis Planning: Our tool employs the Template_relevance Pistachio, Template_relevance Bkms_metabolic, Template_relevance Pistachio_ringbreaker, Template_relevance Reaxys, Template_relevance Reaxys_biocatalysis model, leveraging a vast database of chemical reactions to predict feasible synthetic routes.
One-Step Synthesis Focus: Specifically designed for one-step synthesis, it provides concise and direct routes for your target compounds, streamlining the synthesis process.
Accurate Predictions: Utilizing the extensive PISTACHIO, BKMS_METABOLIC, PISTACHIO_RINGBREAKER, REAXYS, REAXYS_BIOCATALYSIS database, our tool offers high-accuracy predictions, reflecting the latest in chemical research and data.
Strategy Settings
Precursor scoring | Relevance Heuristic |
---|---|
Min. plausibility | 0.01 |
Model | Template_relevance |
Template Set | Pistachio/Bkms_metabolic/Pistachio_ringbreaker/Reaxys/Reaxys_biocatalysis |
Top-N result to add to graph | 6 |
Feasible Synthetic Routes
Avertissement et informations sur les produits de recherche in vitro
Veuillez noter que tous les articles et informations sur les produits présentés sur BenchChem sont destinés uniquement à des fins informatives. Les produits disponibles à l'achat sur BenchChem sont spécifiquement conçus pour des études in vitro, qui sont réalisées en dehors des organismes vivants. Les études in vitro, dérivées du terme latin "in verre", impliquent des expériences réalisées dans des environnements de laboratoire contrôlés à l'aide de cellules ou de tissus. Il est important de noter que ces produits ne sont pas classés comme médicaments et n'ont pas reçu l'approbation de la FDA pour la prévention, le traitement ou la guérison de toute condition médicale, affection ou maladie. Nous devons souligner que toute forme d'introduction corporelle de ces produits chez les humains ou les animaux est strictement interdite par la loi. Il est essentiel de respecter ces directives pour assurer la conformité aux normes légales et éthiques en matière de recherche et d'expérimentation.