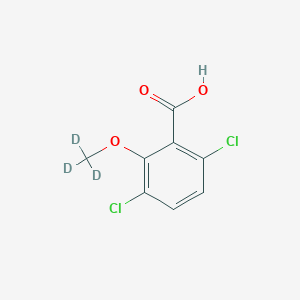
Dicamba-d3
Vue d'ensemble
Description
Dicamba-d3 is a deuterium-labeled derivative of dicamba, which is a widely used herbicide. Dicamba, known chemically as 3,6-dichloro-2-methoxybenzoic acid, has been employed for over 50 years to control broadleaf weeds in various agricultural settings. The deuterium labeling in this compound makes it particularly useful in scientific research, especially in studies involving mass spectrometry and environmental monitoring .
Mécanisme D'action
Target of Action
Dicamba-d3, like its parent compound dicamba, is an auxin-based herbicide . It primarily targets broadleaf weeds , disrupting their normal growth patterns . It is particularly effective against glyphosate-resistant weeds, such as Palmer amaranth (Amaranthus palmeri S. Watson), waterhemp [Amaranthus tuberculatus (Moq.) J. D. Sauer], and horseweed (Erigeron canadensis) .
Mode of Action
This compound acts by mimicking the plant hormone auxin , leading to abnormal growth patterns in target plants . This irregular growth disrupts cell transport systems, which can induce plant death . The compound’s effectiveness is enhanced when applied in combination with other herbicides .
Biochemical Pathways
This compound affects the auxin signaling pathway in plants . It manipulates plant phytohormone responses, specifically increasing ethylene and abscisic acid production . This leads to plant growth inhibition, senescence, and tissue decay . Three primary dicamba metabolites have been identified in the environment: 5-OH-dicamba, 3,6-dichlorosalicylic acid (DCSA), and a secondary metabolite of DCSA, DCGA (3,6-dichlorogentisic acid) .
Pharmacokinetics
This compound is used as an analytical reference standard for the determination of the analyte in fresh water and air samples . It is detected in complex environmental matrices like soil and plant tissues . .
Result of Action
The molecular and cellular effects of this compound action result in disordered growth and eventual death of the target plants . Non-target plants exposed to even small amounts of dicamba may experience injury effects with deformities .
Action Environment
Environmental factors significantly influence the action, efficacy, and stability of this compound. For instance, dicamba volatility can be strongly affected by spray solution pH levels and the presence of certain ions . Regulations have been introduced to control migration, such as restrictions on the time of day for application and conditions to avoid, like high winds .
Analyse Biochimique
Biochemical Properties
Dicamba-d3, like its parent compound Dicamba, is a synthetic auxin . Auxins are a class of plant hormones that play a crucial role in the coordination of many growth and behavioral processes in plants’ life cycles. This compound, therefore, interacts with the same enzymes, proteins, and other biomolecules that natural auxins do . The nature of these interactions is primarily regulatory, influencing the rate of plant growth and leading to the senescence and death of the plant .
Cellular Effects
At the cellular level, this compound can have profound effects. It is known to cause DNA damage levels and changes in DNA methylation . These changes can influence cell function significantly, including impacts on cell signaling pathways, gene expression, and cellular metabolism . The effects of this compound are particularly pronounced in non-resistant crops and other plants .
Molecular Mechanism
The molecular mechanism of this compound is similar to that of Dicamba. As a synthetic auxin, it mimics the action of natural auxins by binding to the auxin receptors in the plant cells . This binding triggers a cascade of events, including the activation or inhibition of certain enzymes, changes in gene expression, and alterations in cellular metabolism .
Temporal Effects in Laboratory Settings
In laboratory settings, the effects of this compound can change over time. For instance, studies have shown that this compound causes DNA damage and changes in DNA methylation . These effects decrease after treatment with humic acids, indicating that the compound’s impact on cellular function can vary over time .
Dosage Effects in Animal Models
While most studies on this compound have focused on its effects on plants, some research has also been conducted on its impact on animals. For instance, a study by the Food and Agriculture Organization found that Dicamba has a low acute toxicity in animals, with the lowest oral median lethal dose being approximately 1600 mg/kg in female rats .
Metabolic Pathways
This compound is involved in several metabolic pathways. It is poorly metabolized, and the pathways involved include demethylation, hydroxylation, and glucuronic acid conjugation . More than 95% of the administered dose is excreted in the urine, with less than 5% in the feces .
Transport and Distribution
This compound, like Dicamba, does not always stay where it is applied. It can migrate via spray drift from the fields where it is intentionally applied, to nearby fields and farms . This indicates that this compound can be transported and distributed within and between different environments.
Subcellular Localization
Given its similarity to Dicamba, it is likely that this compound also accumulates in the meristematic tissues of plants following both foliar and root uptake
Méthodes De Préparation
Synthetic Routes and Reaction Conditions
The synthesis of Dicamba-d3 involves the incorporation of deuterium atoms into the dicamba molecule. This can be achieved through various methods, including the use of deuterated reagents in the synthesis process. One common approach is the deuterium exchange reaction, where hydrogen atoms in the dicamba molecule are replaced with deuterium atoms under specific conditions .
Industrial Production Methods
Industrial production of this compound typically involves large-scale synthesis using deuterated reagents. The process is optimized to ensure high yield and purity of the final product. The use of advanced chromatographic techniques, such as liquid chromatography coupled with mass spectrometry, is essential for the purification and quantification of this compound .
Analyse Des Réactions Chimiques
Types of Reactions
Dicamba-d3, like its non-deuterated counterpart, undergoes various chemical reactions, including:
Oxidation: this compound can be oxidized to form hydroxylated derivatives.
Reduction: Reduction reactions can convert this compound to its corresponding alcohol.
Substitution: Halogen atoms in this compound can be substituted with other functional groups under appropriate conditions.
Common Reagents and Conditions
Oxidation: Common oxidizing agents include potassium permanganate and hydrogen peroxide.
Reduction: Reducing agents such as lithium aluminum hydride are used.
Substitution: Nucleophilic substitution reactions often involve reagents like sodium hydroxide or potassium carbonate.
Major Products Formed
The major products formed from these reactions include hydroxylated dicamba derivatives, dicamba alcohols, and various substituted dicamba compounds .
Applications De Recherche Scientifique
Dicamba-d3 is extensively used in scientific research due to its unique properties:
Chemistry: It serves as an internal standard in mass spectrometry for the quantification of dicamba and its metabolites.
Biology: this compound is used in studies investigating the environmental fate and transport of herbicides.
Medicine: Research on the potential health effects of herbicide exposure often employs this compound as a tracer.
Industry: It is used in the development and validation of analytical methods for herbicide detection in environmental and agricultural samples
Comparaison Avec Des Composés Similaires
Similar Compounds
2,4-Dichlorophenoxyacetic acid (2,4-D): Another widely used herbicide with similar auxin-mimicking properties.
Triclopyr: A herbicide used for woody plant control, also mimicking auxin.
Uniqueness of Dicamba-d3
This compound’s uniqueness lies in its deuterium labeling, which enhances its utility in analytical chemistry. The deuterium atoms provide a distinct mass difference, making it an ideal internal standard for mass spectrometry. This property is particularly valuable in environmental monitoring and residue analysis, where precise quantification is crucial .
Propriétés
IUPAC Name |
3,6-dichloro-2-(trideuteriomethoxy)benzoic acid | |
---|---|---|
Source | PubChem | |
URL | https://pubchem.ncbi.nlm.nih.gov | |
Description | Data deposited in or computed by PubChem | |
InChI |
InChI=1S/C8H6Cl2O3/c1-13-7-5(10)3-2-4(9)6(7)8(11)12/h2-3H,1H3,(H,11,12)/i1D3 | |
Source | PubChem | |
URL | https://pubchem.ncbi.nlm.nih.gov | |
Description | Data deposited in or computed by PubChem | |
InChI Key |
IWEDIXLBFLAXBO-FIBGUPNXSA-N | |
Source | PubChem | |
URL | https://pubchem.ncbi.nlm.nih.gov | |
Description | Data deposited in or computed by PubChem | |
Canonical SMILES |
COC1=C(C=CC(=C1C(=O)O)Cl)Cl | |
Source | PubChem | |
URL | https://pubchem.ncbi.nlm.nih.gov | |
Description | Data deposited in or computed by PubChem | |
Isomeric SMILES |
[2H]C([2H])([2H])OC1=C(C=CC(=C1C(=O)O)Cl)Cl | |
Source | PubChem | |
URL | https://pubchem.ncbi.nlm.nih.gov | |
Description | Data deposited in or computed by PubChem | |
Molecular Formula |
C8H6Cl2O3 | |
Source | PubChem | |
URL | https://pubchem.ncbi.nlm.nih.gov | |
Description | Data deposited in or computed by PubChem | |
DSSTOX Substance ID |
DTXSID40512157 | |
Record name | 3,6-Dichloro-2-[(~2~H_3_)methyloxy]benzoic acid | |
Source | EPA DSSTox | |
URL | https://comptox.epa.gov/dashboard/DTXSID40512157 | |
Description | DSSTox provides a high quality public chemistry resource for supporting improved predictive toxicology. | |
Molecular Weight |
224.05 g/mol | |
Source | PubChem | |
URL | https://pubchem.ncbi.nlm.nih.gov | |
Description | Data deposited in or computed by PubChem | |
CAS No. |
349553-95-3 | |
Record name | 3,6-Dichloro-2-[(~2~H_3_)methyloxy]benzoic acid | |
Source | EPA DSSTox | |
URL | https://comptox.epa.gov/dashboard/DTXSID40512157 | |
Description | DSSTox provides a high quality public chemistry resource for supporting improved predictive toxicology. | |
Record name | Dicamba-D3 | |
Source | European Chemicals Agency (ECHA) | |
URL | https://echa.europa.eu/information-on-chemicals | |
Description | The European Chemicals Agency (ECHA) is an agency of the European Union which is the driving force among regulatory authorities in implementing the EU's groundbreaking chemicals legislation for the benefit of human health and the environment as well as for innovation and competitiveness. | |
Explanation | Use of the information, documents and data from the ECHA website is subject to the terms and conditions of this Legal Notice, and subject to other binding limitations provided for under applicable law, the information, documents and data made available on the ECHA website may be reproduced, distributed and/or used, totally or in part, for non-commercial purposes provided that ECHA is acknowledged as the source: "Source: European Chemicals Agency, http://echa.europa.eu/". Such acknowledgement must be included in each copy of the material. ECHA permits and encourages organisations and individuals to create links to the ECHA website under the following cumulative conditions: Links can only be made to webpages that provide a link to the Legal Notice page. | |
Retrosynthesis Analysis
AI-Powered Synthesis Planning: Our tool employs the Template_relevance Pistachio, Template_relevance Bkms_metabolic, Template_relevance Pistachio_ringbreaker, Template_relevance Reaxys, Template_relevance Reaxys_biocatalysis model, leveraging a vast database of chemical reactions to predict feasible synthetic routes.
One-Step Synthesis Focus: Specifically designed for one-step synthesis, it provides concise and direct routes for your target compounds, streamlining the synthesis process.
Accurate Predictions: Utilizing the extensive PISTACHIO, BKMS_METABOLIC, PISTACHIO_RINGBREAKER, REAXYS, REAXYS_BIOCATALYSIS database, our tool offers high-accuracy predictions, reflecting the latest in chemical research and data.
Strategy Settings
Precursor scoring | Relevance Heuristic |
---|---|
Min. plausibility | 0.01 |
Model | Template_relevance |
Template Set | Pistachio/Bkms_metabolic/Pistachio_ringbreaker/Reaxys/Reaxys_biocatalysis |
Top-N result to add to graph | 6 |
Feasible Synthetic Routes
Avertissement et informations sur les produits de recherche in vitro
Veuillez noter que tous les articles et informations sur les produits présentés sur BenchChem sont destinés uniquement à des fins informatives. Les produits disponibles à l'achat sur BenchChem sont spécifiquement conçus pour des études in vitro, qui sont réalisées en dehors des organismes vivants. Les études in vitro, dérivées du terme latin "in verre", impliquent des expériences réalisées dans des environnements de laboratoire contrôlés à l'aide de cellules ou de tissus. Il est important de noter que ces produits ne sont pas classés comme médicaments et n'ont pas reçu l'approbation de la FDA pour la prévention, le traitement ou la guérison de toute condition médicale, affection ou maladie. Nous devons souligner que toute forme d'introduction corporelle de ces produits chez les humains ou les animaux est strictement interdite par la loi. Il est essentiel de respecter ces directives pour assurer la conformité aux normes légales et éthiques en matière de recherche et d'expérimentation.