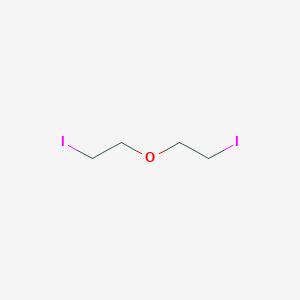
Éther de 2-iodoéthyle
Vue d'ensemble
Description
It is a brown oil with a density of 2.296 g/mL at 25°C and a boiling point of 140-141°C (decomposition) . This compound is primarily used in organic synthesis as a reagent or intermediate.
Applications De Recherche Scientifique
2-Iodoethyl ether has several scientific research applications, including:
Organic Synthesis: It is used as a reagent or intermediate in the synthesis of various organic compounds.
Medicinal Chemistry: It is used in the development of pharmaceuticals and other bioactive compounds.
Material Science: It is used in the synthesis of polymers and other materials with specific properties.
Chemical Biology: It is used in the study of biological processes and the development of chemical probes.
Mécanisme D'action
Target of Action
2-Iodoethyl ether, also known as 1-iodo-2-(2-iodoethoxy)ethane, is primarily used as a laboratory chemical and in the synthesis of substances . .
Biochemical Pathways
Its role in synthesis suggests it may be involved in various chemical reactions, but these would be dependent on the specific substances being synthesized .
Result of Action
As a synthetic chemical, its effects would likely be dependent on the specific substances it is used to synthesize .
Action Environment
Environmental factors can influence the action, efficacy, and stability of 2-Iodoethyl ether. For example, it is combustible and forms explosive mixtures with air on intense heating . Therefore, it should be stored in a well-ventilated place and kept away from heat, sparks, open flames, and hot surfaces .
Méthodes De Préparation
2-Iodoethyl ether can be synthesized through the reaction of ethylene glycol with iodine and a base. The typical synthetic route involves the following steps:
Formation of Ethylene Glycol Diiodide: Ethylene glycol reacts with iodine in the presence of a base such as sodium hydroxide to form ethylene glycol diiodide.
Industrial production methods for 2-Iodoethyl ether are similar to the laboratory synthesis but are scaled up to accommodate larger quantities. The reaction conditions are optimized to ensure high yield and purity of the product.
Analyse Des Réactions Chimiques
2-Iodoethyl ether undergoes various types of chemical reactions, including:
Substitution Reactions: It can undergo nucleophilic substitution reactions where the iodine atoms are replaced by other nucleophiles such as hydroxide ions, amines, or thiols.
Oxidation Reactions: The ether can be oxidized to form corresponding carbonyl compounds.
Reduction Reactions: It can be reduced to form ethyl ether derivatives.
Common reagents and conditions used in these reactions include:
Nucleophiles: Hydroxide ions, amines, thiols.
Oxidizing Agents: Potassium permanganate, chromium trioxide.
Reducing Agents: Lithium aluminum hydride, sodium borohydride.
Major products formed from these reactions include substituted ethers, carbonyl compounds, and reduced ether derivatives .
Comparaison Avec Des Composés Similaires
2-Iodoethyl ether can be compared with other similar compounds such as:
2-Bromoethyl ether: Similar in structure but contains bromine instead of iodine. It is less reactive due to the lower leaving group ability of bromine compared to iodine.
2-Chloroethyl ether: Contains chlorine instead of iodine. It is even less reactive than 2-Bromoethyl ether due to the poor leaving group ability of chlorine.
2-Fluoroethyl ether: Contains fluorine instead of iodine. It is the least reactive among the halogenated ethyl ethers due to the very poor leaving group ability of fluorine.
The uniqueness of 2-Iodoethyl ether lies in its high reactivity due to the presence of iodine, which is an excellent leaving group. This makes it particularly useful in organic synthesis for the formation of various substituted products .
Activité Biologique
2-Iodoethyl ether is an organoiodine compound with the formula C4H8I2O. Its biological activity has garnered interest in various fields, particularly in medicinal chemistry and virology. This article provides a detailed overview of the biological activity of 2-Iodoethyl ether, including its synthesis, mechanisms of action, and relevant case studies.
2-Iodoethyl ether is characterized by its iodine content, which contributes to its biological activity. The synthesis of this compound typically involves the reaction of ethylene oxide with iodine or iodoethanol under controlled conditions. For instance, one study described a method where iodomethoxy derivatives were synthesized through the addition of iodine monochloride to vinyl moieties in the presence of alcohols .
The biological activity of 2-Iodoethyl ether is primarily linked to its antiviral properties. It has been shown to inhibit the replication of certain viruses by interfering with their ability to produce viral proteins. For example, in studies involving Sindbis virus (SINV), 2-Iodoethyl ether demonstrated significant antiviral activity, with effective concentrations (EC50) established through cell viability assays .
Table 1: Antiviral Activity of 2-Iodoethyl Ether
Compound | EC50 (µM) | Virus Type |
---|---|---|
2-Iodoethyl Ether | 14 | Sindbis Virus |
Dioxane-based derivative | 14 | Sindbis Virus |
Cytotoxicity Studies
Cytotoxicity assays are crucial for evaluating the safety profile of any potential therapeutic agent. In experiments conducted on baby hamster kidney (BHK) cells, various concentrations of 2-Iodoethyl ether were tested. The results indicated that while the compound exhibited antiviral properties, it also displayed cytotoxic effects at higher concentrations. The XTT-based colorimetric assay was employed to determine cell viability, revealing a dose-dependent relationship between compound concentration and cell survival .
Case Studies and Applications
- Antiviral Applications : A notable study focused on the use of 2-Iodoethyl ether in treating viral infections. The compound was tested against SINV, showing promising results in inhibiting viral replication without excessive toxicity to host cells .
- Medical Applications : Historical records indicate that iodine compounds like 2-Iodoethyl ether have been used for their antiseptic properties in treating pulmonary infections. Its effectiveness was noted in cases such as bronchiectasis and pneumonia, where it was used as part of a therapeutic regimen .
- Research on Derivatives : Further investigations into modified derivatives of 2-Iodoethyl ether have been conducted to enhance its biological activity and reduce toxicity. For instance, researchers have explored alkoxyhalogenalkyl derivatives for improved selectivity against viral targets while minimizing cytotoxicity .
Propriétés
IUPAC Name |
1-iodo-2-(2-iodoethoxy)ethane | |
---|---|---|
Source | PubChem | |
URL | https://pubchem.ncbi.nlm.nih.gov | |
Description | Data deposited in or computed by PubChem | |
InChI |
InChI=1S/C4H8I2O/c5-1-3-7-4-2-6/h1-4H2 | |
Source | PubChem | |
URL | https://pubchem.ncbi.nlm.nih.gov | |
Description | Data deposited in or computed by PubChem | |
InChI Key |
XWYDQETVMJZUOJ-UHFFFAOYSA-N | |
Source | PubChem | |
URL | https://pubchem.ncbi.nlm.nih.gov | |
Description | Data deposited in or computed by PubChem | |
Canonical SMILES |
C(CI)OCCI | |
Source | PubChem | |
URL | https://pubchem.ncbi.nlm.nih.gov | |
Description | Data deposited in or computed by PubChem | |
Molecular Formula |
C4H8I2O | |
Source | PubChem | |
URL | https://pubchem.ncbi.nlm.nih.gov | |
Description | Data deposited in or computed by PubChem | |
DSSTOX Substance ID |
DTXSID30392405 | |
Record name | 2-Iodoethyl ether | |
Source | EPA DSSTox | |
URL | https://comptox.epa.gov/dashboard/DTXSID30392405 | |
Description | DSSTox provides a high quality public chemistry resource for supporting improved predictive toxicology. | |
Molecular Weight |
325.91 g/mol | |
Source | PubChem | |
URL | https://pubchem.ncbi.nlm.nih.gov | |
Description | Data deposited in or computed by PubChem | |
CAS No. |
34270-90-1 | |
Record name | 2-Iodoethyl ether | |
Source | EPA DSSTox | |
URL | https://comptox.epa.gov/dashboard/DTXSID30392405 | |
Description | DSSTox provides a high quality public chemistry resource for supporting improved predictive toxicology. | |
Record name | 2-Iodoethyl ether | |
Source | European Chemicals Agency (ECHA) | |
URL | https://echa.europa.eu/information-on-chemicals | |
Description | The European Chemicals Agency (ECHA) is an agency of the European Union which is the driving force among regulatory authorities in implementing the EU's groundbreaking chemicals legislation for the benefit of human health and the environment as well as for innovation and competitiveness. | |
Explanation | Use of the information, documents and data from the ECHA website is subject to the terms and conditions of this Legal Notice, and subject to other binding limitations provided for under applicable law, the information, documents and data made available on the ECHA website may be reproduced, distributed and/or used, totally or in part, for non-commercial purposes provided that ECHA is acknowledged as the source: "Source: European Chemicals Agency, http://echa.europa.eu/". Such acknowledgement must be included in each copy of the material. ECHA permits and encourages organisations and individuals to create links to the ECHA website under the following cumulative conditions: Links can only be made to webpages that provide a link to the Legal Notice page. | |
Retrosynthesis Analysis
AI-Powered Synthesis Planning: Our tool employs the Template_relevance Pistachio, Template_relevance Bkms_metabolic, Template_relevance Pistachio_ringbreaker, Template_relevance Reaxys, Template_relevance Reaxys_biocatalysis model, leveraging a vast database of chemical reactions to predict feasible synthetic routes.
One-Step Synthesis Focus: Specifically designed for one-step synthesis, it provides concise and direct routes for your target compounds, streamlining the synthesis process.
Accurate Predictions: Utilizing the extensive PISTACHIO, BKMS_METABOLIC, PISTACHIO_RINGBREAKER, REAXYS, REAXYS_BIOCATALYSIS database, our tool offers high-accuracy predictions, reflecting the latest in chemical research and data.
Strategy Settings
Precursor scoring | Relevance Heuristic |
---|---|
Min. plausibility | 0.01 |
Model | Template_relevance |
Template Set | Pistachio/Bkms_metabolic/Pistachio_ringbreaker/Reaxys/Reaxys_biocatalysis |
Top-N result to add to graph | 6 |
Feasible Synthetic Routes
Q1: What is the primary use of 2-iodoethyl ether in chemical synthesis?
A: 2-Iodoethyl ether is commonly employed as an alkylating agent in organic synthesis. Its primary function is to introduce the ethoxyethyl group (-CH2CH2OCH2CH2-) into target molecules. This reagent has been particularly useful in constructing macrocyclic compounds, as seen in its application for synthesizing crown ether derivatives [], chiral cryptands from sugar derivatives [], and triazolophanes containing oxygen and sulfur heteroatoms [].
Q2: How does the structure of 2-iodoethyl ether contribute to its reactivity in macrocycle formation?
A: The two iodoethyl groups present in 2-iodoethyl ether allow for a two-step alkylation process, crucial for closing macrocyclic rings. This reagent can react with two nucleophilic centers in a molecule, effectively linking them together through an ethylene bridge. For instance, it reacts with diaza crown ethers, forming quaternary salts that can be further demethylated to yield chiral cryptands [].
Q3: Can you explain the role of 2-iodoethyl ether in the synthesis of 1,3-dithiole-2-thione tetramers?
A: 2-Iodoethyl ether serves as a linker in the multi-step synthesis of crown ether derivative-annulated 1,3-dithiole-2-thione tetramers []. It reacts with 1,3-dithiole-2-thione-4,5-biscyanoethyl, ultimately contributing to the cyclic structure of the target tetramer.
Q4: Are there any studies investigating the potential of 2-iodoethyl ether derivatives in medicinal chemistry?
A: Yes, researchers have explored the use of 2-iodoethyl ether derivatives in synthesizing potential anticancer agents. One study involved using bis(2-iodoethyl) ether to prepare 3'-morpholino-pirarubicin derivatives []. These derivatives exhibited improved antitumor effects in murine tumor models, highlighting the potential of 2-iodoethyl ether-derived compounds in medicinal chemistry.
Q5: Has 2-iodoethyl ether been utilized in coordination chemistry?
A: Research demonstrates the use of 2-iodoethyl ether in synthesizing nickel(II) complexes containing macrocyclic ligands []. In this context, the reaction of a nickel(II) complex with 2-iodoethyl ether led to the formation of a six-coordinate complex. This complex incorporated a pentadentate macrocyclic ligand with N2S2O donor atoms, demonstrating the utility of 2-iodoethyl ether in constructing metal complexes with specific coordination environments.
Q6: What are the analytical techniques used to characterize compounds synthesized using 2-iodoethyl ether?
A6: Various analytical techniques are employed to characterize the compounds synthesized using 2-iodoethyl ether. These include:
- Nuclear Magnetic Resonance (NMR) spectroscopy: This technique provides structural information by analyzing the magnetic properties of atomic nuclei, as demonstrated in studies investigating glycosylation reactions [] and characterizing synthesized 1,3-dithiole-2-thione tetramers [].
- Mass Spectrometry (MS): MS is used to determine the mass-to-charge ratio of ions, providing information about the molecular weight and fragmentation patterns of the synthesized compounds [].
- Elemental Analysis (EA): This technique determines the percentage composition of elements in a compound, confirming its purity and elemental composition [].
- X-ray crystallography: This method is used to determine the three-dimensional structure of crystalline compounds, offering insights into the spatial arrangement of atoms within the molecule, as seen in the analysis of nickel(II) complexes [].
Avertissement et informations sur les produits de recherche in vitro
Veuillez noter que tous les articles et informations sur les produits présentés sur BenchChem sont destinés uniquement à des fins informatives. Les produits disponibles à l'achat sur BenchChem sont spécifiquement conçus pour des études in vitro, qui sont réalisées en dehors des organismes vivants. Les études in vitro, dérivées du terme latin "in verre", impliquent des expériences réalisées dans des environnements de laboratoire contrôlés à l'aide de cellules ou de tissus. Il est important de noter que ces produits ne sont pas classés comme médicaments et n'ont pas reçu l'approbation de la FDA pour la prévention, le traitement ou la guérison de toute condition médicale, affection ou maladie. Nous devons souligner que toute forme d'introduction corporelle de ces produits chez les humains ou les animaux est strictement interdite par la loi. Il est essentiel de respecter ces directives pour assurer la conformité aux normes légales et éthiques en matière de recherche et d'expérimentation.