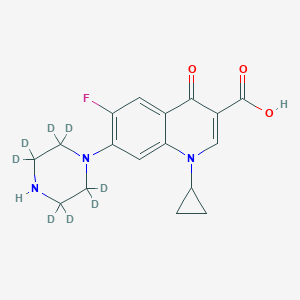
Ciprofloxacine-d8
Vue d'ensemble
Description
Ciprofloxacin-d8 is a deuterium-labeled derivative of ciprofloxacin, a fluoroquinolone antibiotic. This compound is used primarily as an internal standard in various analytical methods, particularly in mass spectrometry, to quantify ciprofloxacin levels in biological and environmental samples .
Applications De Recherche Scientifique
Ciprofloxacin-d8 is widely used in scientific research due to its stable isotopic labeling. Some key applications include:
Analytical Chemistry: Used as an internal standard in mass spectrometry for the quantification of ciprofloxacin in biological and environmental samples.
Pharmacokinetics: Helps in studying the pharmacokinetics and metabolism of ciprofloxacin by providing accurate quantification.
Environmental Studies: Used to monitor the presence and degradation of ciprofloxacin in environmental samples.
Biomedical Research: Assists in understanding the distribution and excretion of ciprofloxacin in various biological systems .
Mécanisme D'action
Target of Action
Ciprofloxacin-d8, a deuterium-labeled variant of Ciprofloxacin, primarily targets two enzymes: bacterial DNA gyrase and topoisomerase IV . These enzymes play a crucial role in DNA replication and repair, which are essential for bacterial growth and survival .
Mode of Action
Ciprofloxacin-d8 interacts with its targets by inhibiting the activity of DNA gyrase and topoisomerase IV . This inhibition prevents the supercoiling of bacterial DNA, which is a critical step in DNA replication . As a result, the replication of bacterial DNA is hampered, leading to the cessation of bacterial cell growth .
Biochemical Pathways
The primary biochemical pathway affected by Ciprofloxacin-d8 is the DNA replication pathway in bacteria . By inhibiting DNA gyrase and topoisomerase IV, Ciprofloxacin-d8 disrupts the supercoiling process, a crucial step in DNA replication . This disruption leads to the cessation of bacterial DNA replication, thereby inhibiting bacterial growth .
Result of Action
The primary result of Ciprofloxacin-d8’s action is the inhibition of bacterial growth . By preventing DNA replication, Ciprofloxacin-d8 effectively hampers bacterial replication, ultimately leading to bacterial death . This makes it a potent antibacterial agent, particularly against Gram-negative bacteria .
Action Environment
The action of Ciprofloxacin-d8 can be influenced by various environmental factors. For instance, the presence of other carbon or nitrogen sources can enhance the biodegradation of Ciprofloxacin . Furthermore, the compound’s stability and efficacy can be affected by factors such as temperature, pH, and the presence of other substances in the environment . .
Analyse Biochimique
Biochemical Properties
Ciprofloxacin-d8, like its parent compound ciprofloxacin, interacts with various enzymes and proteins. It primarily targets DNA gyrase and topoisomerase IV, which are essential enzymes for bacterial DNA replication . By inhibiting these enzymes, ciprofloxacin-d8 prevents bacterial DNA replication, leading to the death of the bacteria .
Cellular Effects
Ciprofloxacin-d8 has significant effects on cellular processes. It has been shown to cause a decrease in cell densities and chlorophyll-a in certain strains of bacteria . Additionally, it has been found to alter the normal maintenance and transcription of mitochondrial DNA, leading to impaired mitochondrial energy production and blocking cellular growth .
Molecular Mechanism
The molecular mechanism of action of ciprofloxacin-d8 is similar to that of ciprofloxacin. It inhibits DNA gyrase and topoisomerase IV, enzymes crucial for bacterial DNA replication . This inhibition prevents the bacteria from replicating their DNA, thereby stopping their growth and leading to their death .
Temporal Effects in Laboratory Settings
In laboratory settings, the effects of ciprofloxacin-d8 have been observed to change over time. For instance, it has been used in liquid chromatography-tandem mass spectrometric (LC-MS/MS) methods for determining ciprofloxacin in small sample volumes of plasma, urine, bladder, and kidneys of mice .
Metabolic Pathways
Ciprofloxacin-d8 is involved in several metabolic pathways. It is known to be a substrate of P-glycoprotein (ABCB1), which plays a significant role in drug metabolism
Transport and Distribution
The transport and distribution of ciprofloxacin-d8 within cells and tissues are influenced by various factors. For instance, the presence of multi-walled carbon nanotubes in saturated porous media significantly inhibited the transport of ciprofloxacin-d8 .
Subcellular Localization
Given its molecular mechanism of action, it is likely to localize in the regions where DNA gyrase and topoisomerase IV are found, which is in the bacterial cytoplasm .
Méthodes De Préparation
Synthetic Routes and Reaction Conditions: The synthesis of ciprofloxacin-d8 involves the incorporation of deuterium atoms into the ciprofloxacin molecule. This is typically achieved through the use of deuterated reagents or solvents during the synthesis process. The key steps include:
Cyclopropylamine Reaction: The reaction of cyclopropylamine with a fluorinated quinolone intermediate.
Deuterium Exchange:
Industrial Production Methods: Industrial production of ciprofloxacin-d8 follows similar synthetic routes but on a larger scale. The process involves stringent quality control measures to ensure the purity and isotopic enrichment of the final product .
Analyse Des Réactions Chimiques
Types of Reactions: Ciprofloxacin-d8 undergoes various chemical reactions, including:
Oxidation: The oxidation of ciprofloxacin-d8 can occur under specific conditions, leading to the formation of oxo-derivatives.
Reduction: Reduction reactions can convert ciprofloxacin-d8 into its reduced forms.
Substitution: Substitution reactions can occur at the piperazine ring or the quinolone core.
Common Reagents and Conditions:
Oxidation: Common oxidizing agents include hydrogen peroxide and potassium permanganate.
Reduction: Reducing agents such as sodium borohydride are used.
Substitution: Various nucleophiles can be used for substitution reactions under controlled conditions.
Major Products: The major products formed from these reactions depend on the specific reagents and conditions used. For example, oxidation can lead to the formation of oxo-derivatives, while reduction can yield reduced forms of ciprofloxacin-d8 .
Comparaison Avec Des Composés Similaires
Enrofloxacin-d5: Another deuterium-labeled fluoroquinolone used as an internal standard.
Ofloxacin-d3: A deuterium-labeled derivative of ofloxacin, used in similar analytical applications.
Uniqueness: Ciprofloxacin-d8 is unique due to its specific labeling pattern, which provides distinct mass spectrometric properties. This makes it highly suitable for accurate quantification in complex matrices. Additionally, its widespread use in various fields of research highlights its versatility and importance .
Propriétés
IUPAC Name |
1-cyclopropyl-6-fluoro-7-(2,2,3,3,5,5,6,6-octadeuteriopiperazin-1-yl)-4-oxoquinoline-3-carboxylic acid | |
---|---|---|
Source | PubChem | |
URL | https://pubchem.ncbi.nlm.nih.gov | |
Description | Data deposited in or computed by PubChem | |
InChI |
InChI=1S/C17H18FN3O3/c18-13-7-11-14(8-15(13)20-5-3-19-4-6-20)21(10-1-2-10)9-12(16(11)22)17(23)24/h7-10,19H,1-6H2,(H,23,24)/i3D2,4D2,5D2,6D2 | |
Source | PubChem | |
URL | https://pubchem.ncbi.nlm.nih.gov | |
Description | Data deposited in or computed by PubChem | |
InChI Key |
MYSWGUAQZAJSOK-SQUIKQQTSA-N | |
Source | PubChem | |
URL | https://pubchem.ncbi.nlm.nih.gov | |
Description | Data deposited in or computed by PubChem | |
Canonical SMILES |
C1CC1N2C=C(C(=O)C3=CC(=C(C=C32)N4CCNCC4)F)C(=O)O | |
Source | PubChem | |
URL | https://pubchem.ncbi.nlm.nih.gov | |
Description | Data deposited in or computed by PubChem | |
Isomeric SMILES |
[2H]C1(C(N(C(C(N1)([2H])[2H])([2H])[2H])C2=C(C=C3C(=C2)N(C=C(C3=O)C(=O)O)C4CC4)F)([2H])[2H])[2H] | |
Source | PubChem | |
URL | https://pubchem.ncbi.nlm.nih.gov | |
Description | Data deposited in or computed by PubChem | |
Molecular Formula |
C17H18FN3O3 | |
Source | PubChem | |
URL | https://pubchem.ncbi.nlm.nih.gov | |
Description | Data deposited in or computed by PubChem | |
DSSTOX Substance ID |
DTXSID40649159 | |
Record name | Ciprofloxacin-d8 | |
Source | EPA DSSTox | |
URL | https://comptox.epa.gov/dashboard/DTXSID40649159 | |
Description | DSSTox provides a high quality public chemistry resource for supporting improved predictive toxicology. | |
Molecular Weight |
339.39 g/mol | |
Source | PubChem | |
URL | https://pubchem.ncbi.nlm.nih.gov | |
Description | Data deposited in or computed by PubChem | |
CAS No. |
1130050-35-9 | |
Record name | Ciprofloxacin-d8 | |
Source | EPA DSSTox | |
URL | https://comptox.epa.gov/dashboard/DTXSID40649159 | |
Description | DSSTox provides a high quality public chemistry resource for supporting improved predictive toxicology. | |
Synthesis routes and methods I
Procedure details
Synthesis routes and methods II
Procedure details
Synthesis routes and methods III
Procedure details
Synthesis routes and methods IV
Procedure details
Retrosynthesis Analysis
AI-Powered Synthesis Planning: Our tool employs the Template_relevance Pistachio, Template_relevance Bkms_metabolic, Template_relevance Pistachio_ringbreaker, Template_relevance Reaxys, Template_relevance Reaxys_biocatalysis model, leveraging a vast database of chemical reactions to predict feasible synthetic routes.
One-Step Synthesis Focus: Specifically designed for one-step synthesis, it provides concise and direct routes for your target compounds, streamlining the synthesis process.
Accurate Predictions: Utilizing the extensive PISTACHIO, BKMS_METABOLIC, PISTACHIO_RINGBREAKER, REAXYS, REAXYS_BIOCATALYSIS database, our tool offers high-accuracy predictions, reflecting the latest in chemical research and data.
Strategy Settings
Precursor scoring | Relevance Heuristic |
---|---|
Min. plausibility | 0.01 |
Model | Template_relevance |
Template Set | Pistachio/Bkms_metabolic/Pistachio_ringbreaker/Reaxys/Reaxys_biocatalysis |
Top-N result to add to graph | 6 |
Feasible Synthetic Routes
Q1: Why is Ciprofloxacin-d8 used as an internal standard for ciprofloxacin analysis?
A1: Ciprofloxacin-d8 shares almost identical chemical properties with ciprofloxacin, except for the eight deuterium atoms replacing hydrogens. This isotopic substitution leads to a slightly different mass, detectable by mass spectrometry, while maintaining similar chromatographic behavior. Using ciprofloxacin-d8 as an internal standard corrects for variations during sample preparation and analysis, improving the accuracy and reliability of ciprofloxacin quantification. [, , , ]
Q2: In what types of samples has ciprofloxacin-d8 been used for ciprofloxacin quantification?
A2: The provided research articles demonstrate the use of ciprofloxacin-d8 for ciprofloxacin analysis in a diverse range of matrices, including:
- Food Products: Honey []
- Biological Samples: Fish and shellfish tissues [], human serum, and urine []
- Environmental Samples: Water from fee-fishing ponds []
Q3: Can you elaborate on the analytical methods employed in conjunction with ciprofloxacin-d8 for ciprofloxacin analysis?
A3: The research primarily utilizes High-Performance Liquid Chromatography coupled with Tandem Mass Spectrometry (HPLC-MS/MS) for ciprofloxacin analysis. This technique involves:
- Extraction: Ciprofloxacin is extracted from the sample, often using solvents and solid-phase extraction techniques. Ciprofloxacin-d8 is added during this step. [, , , ]
- Separation: The extracted sample is separated based on chemical properties using HPLC. [, , , ]
- Detection & Quantification: The separated compounds are detected using mass spectrometry, which can differentiate ciprofloxacin from ciprofloxacin-d8 based on mass differences. The ratio of their signals is then used to accurately calculate the ciprofloxacin concentration. [, , , ]
Q4: Are there specific regulations or guidelines regarding the use of ciprofloxacin-d8 in analytical methods?
A4: While specific regulations might vary between countries and agencies, analytical methods employing internal standards like ciprofloxacin-d8 should adhere to established guidelines for method validation. This typically includes demonstrating acceptable accuracy, precision, linearity, recovery, and limits of detection and quantification. [, , , ]
Q5: The research mentions concerns about antibiotic residues. Why is the presence of ciprofloxacin in these samples a concern?
A5: The detection of ciprofloxacin in food products like honey [], fish [], and environmental water samples [] raises concerns due to the potential for:
- Antibiotic Resistance: The presence of antibiotic residues in the environment and food chain contributes to the development and spread of antibiotic-resistant bacteria, posing a significant threat to human health. [, , ]
- Human Health Risks: Consuming contaminated food could lead to unintended antibiotic exposure, potentially triggering allergic reactions, disrupting gut microbiota, or contributing to antibiotic resistance in individuals. [, , ]
Avertissement et informations sur les produits de recherche in vitro
Veuillez noter que tous les articles et informations sur les produits présentés sur BenchChem sont destinés uniquement à des fins informatives. Les produits disponibles à l'achat sur BenchChem sont spécifiquement conçus pour des études in vitro, qui sont réalisées en dehors des organismes vivants. Les études in vitro, dérivées du terme latin "in verre", impliquent des expériences réalisées dans des environnements de laboratoire contrôlés à l'aide de cellules ou de tissus. Il est important de noter que ces produits ne sont pas classés comme médicaments et n'ont pas reçu l'approbation de la FDA pour la prévention, le traitement ou la guérison de toute condition médicale, affection ou maladie. Nous devons souligner que toute forme d'introduction corporelle de ces produits chez les humains ou les animaux est strictement interdite par la loi. Il est essentiel de respecter ces directives pour assurer la conformité aux normes légales et éthiques en matière de recherche et d'expérimentation.