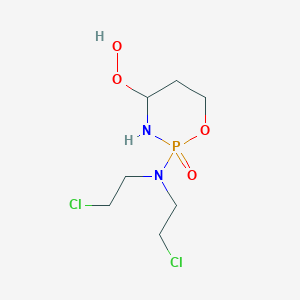
4-Hydroperoxycyclophosphamide
Vue d'ensemble
Description
4-Hydroperoxycyclophosphamide is the active metabolite form of the prodrug Cyclophosphamide . It crosslinks DNA and induces T cell apoptosis independent of death receptor activation, but activates mitochondrial death pathways through the production of reactive oxygen species (ROS) . It is used to treat lymphomas and autoimmune disorders .
Molecular Structure Analysis
The molecular formula of 4-Hydroperoxycyclophosphamide is C7H15Cl2N2O4P . Its average mass is 293.085 Da and its monoisotopic mass is 292.014648 Da .
Applications De Recherche Scientifique
Anti-Cancer Prodrug
4-Hydroperoxycyclophosphamide is a widely used anti-cancer prodrug . It is converted by liver cytochrome P450 (CYP) enzymes into the primary metabolite 4-hydroxycyclophosphamide (4-OH-CPA), followed by non-enzymatic generation of the bioactive metabolites phosphoramide mustard and acrolein .
Toxicological Studies
The compound is used in toxicological studies on human cancer cells . The use of human drug metabolites as authentic standards to evaluate their toxicity is essential for drug development .
Biocatalyst for Selective Cyclophosphamide Hydroxylation
Unspecific peroxygenase from Marasmius rotula serves as an efficient biocatalyst for selective cyclophosphamide hydroxylation . This process leads to the production of 4-hydroxycyclophosphamide .
Cyto- and Genotoxicity Evaluation
4-Hydroperoxycyclophosphamide can be used for direct cyto- and genotoxicity evaluation in human cancer cells . This is important for understanding the impact of the compound on cellular health and integrity .
Interstitial Chemotherapy Delivery
4-Hydroperoxycyclophosphamide is used in interstitial chemotherapy delivery . High drug concentrations were measured within the first 3 mm from the polymer implant; significant concentrations were measured up to ∼5 cm from the implant as long as 30 days after implantation .
Pharmacokinetic Framework
The compound is used in the development of a pharmacokinetic framework for application of a new modality of chemotherapy delivery in primates .
Safety And Hazards
Orientations Futures
Future research could focus on the cardiotoxic mechanisms of cyclophosphamide and its main biotransformation products, including 4-Hydroperoxycyclophosphamide . Another potential area of study could be the use of unspecific peroxygenase from Marasmius rotula as an efficient biocatalyst for selective cyclophosphamide hydroxylation .
Propriétés
IUPAC Name |
N,N-bis(2-chloroethyl)-4-hydroperoxy-2-oxo-1,3,2λ5-oxazaphosphinan-2-amine | |
---|---|---|
Source | PubChem | |
URL | https://pubchem.ncbi.nlm.nih.gov | |
Description | Data deposited in or computed by PubChem | |
InChI |
InChI=1S/C7H15Cl2N2O4P/c8-2-4-11(5-3-9)16(13)10-7(15-12)1-6-14-16/h7,12H,1-6H2,(H,10,13) | |
Source | PubChem | |
URL | https://pubchem.ncbi.nlm.nih.gov | |
Description | Data deposited in or computed by PubChem | |
InChI Key |
VPAWVRUHMJVRHU-UHFFFAOYSA-N | |
Source | PubChem | |
URL | https://pubchem.ncbi.nlm.nih.gov | |
Description | Data deposited in or computed by PubChem | |
Canonical SMILES |
C1COP(=O)(NC1OO)N(CCCl)CCCl | |
Source | PubChem | |
URL | https://pubchem.ncbi.nlm.nih.gov | |
Description | Data deposited in or computed by PubChem | |
Molecular Formula |
C7H15Cl2N2O4P | |
Source | PubChem | |
URL | https://pubchem.ncbi.nlm.nih.gov | |
Description | Data deposited in or computed by PubChem | |
DSSTOX Substance ID |
DTXSID2049058 | |
Record name | 4-Hydroperoxy cyclophosphamide | |
Source | EPA DSSTox | |
URL | https://comptox.epa.gov/dashboard/DTXSID2049058 | |
Description | DSSTox provides a high quality public chemistry resource for supporting improved predictive toxicology. | |
Molecular Weight |
293.08 g/mol | |
Source | PubChem | |
URL | https://pubchem.ncbi.nlm.nih.gov | |
Description | Data deposited in or computed by PubChem | |
Product Name |
4-Hydroperoxycyclophosphamide | |
CAS RN |
39800-16-3 | |
Record name | 4-Hydroperoxycyclophosphamide | |
Source | CAS Common Chemistry | |
URL | https://commonchemistry.cas.org/detail?cas_rn=39800-16-3 | |
Description | CAS Common Chemistry is an open community resource for accessing chemical information. Nearly 500,000 chemical substances from CAS REGISTRY cover areas of community interest, including common and frequently regulated chemicals, and those relevant to high school and undergraduate chemistry classes. This chemical information, curated by our expert scientists, is provided in alignment with our mission as a division of the American Chemical Society. | |
Explanation | The data from CAS Common Chemistry is provided under a CC-BY-NC 4.0 license, unless otherwise stated. | |
Record name | ASTA 6496 | |
Source | ChemIDplus | |
URL | https://pubchem.ncbi.nlm.nih.gov/substance/?source=chemidplus&sourceid=0039800163 | |
Description | ChemIDplus is a free, web search system that provides access to the structure and nomenclature authority files used for the identification of chemical substances cited in National Library of Medicine (NLM) databases, including the TOXNET system. | |
Record name | 4-Hydroperoxycyclophosphamide | |
Source | DTP/NCI | |
URL | https://dtp.cancer.gov/dtpstandard/servlet/dwindex?searchtype=NSC&outputformat=html&searchlist=181815 | |
Description | The NCI Development Therapeutics Program (DTP) provides services and resources to the academic and private-sector research communities worldwide to facilitate the discovery and development of new cancer therapeutic agents. | |
Explanation | Unless otherwise indicated, all text within NCI products is free of copyright and may be reused without our permission. Credit the National Cancer Institute as the source. | |
Record name | 4-Hydroperoxy cyclophosphamide | |
Source | EPA DSSTox | |
URL | https://comptox.epa.gov/dashboard/DTXSID2049058 | |
Description | DSSTox provides a high quality public chemistry resource for supporting improved predictive toxicology. | |
Retrosynthesis Analysis
AI-Powered Synthesis Planning: Our tool employs the Template_relevance Pistachio, Template_relevance Bkms_metabolic, Template_relevance Pistachio_ringbreaker, Template_relevance Reaxys, Template_relevance Reaxys_biocatalysis model, leveraging a vast database of chemical reactions to predict feasible synthetic routes.
One-Step Synthesis Focus: Specifically designed for one-step synthesis, it provides concise and direct routes for your target compounds, streamlining the synthesis process.
Accurate Predictions: Utilizing the extensive PISTACHIO, BKMS_METABOLIC, PISTACHIO_RINGBREAKER, REAXYS, REAXYS_BIOCATALYSIS database, our tool offers high-accuracy predictions, reflecting the latest in chemical research and data.
Strategy Settings
Precursor scoring | Relevance Heuristic |
---|---|
Min. plausibility | 0.01 |
Model | Template_relevance |
Template Set | Pistachio/Bkms_metabolic/Pistachio_ringbreaker/Reaxys/Reaxys_biocatalysis |
Top-N result to add to graph | 6 |
Feasible Synthetic Routes
Q & A
Q1: How does 4-hydroperoxycyclophosphamide exert its antitumor activity?
A1: 4-hydroperoxycyclophosphamide (4-OOH-CPA) is an activated analog of the alkylating agent cyclophosphamide. Unlike cyclophosphamide, 4-OOH-CPA does not require metabolic activation by the liver and readily breaks down into its active metabolites, primarily phosphoramide mustard and acrolein. [, ] Phosphoramide mustard is a potent DNA alkylating agent, forming crosslinks between DNA strands. [, ] This DNA damage disrupts essential cellular processes like replication and transcription, ultimately leading to cell death. [, ]
Q2: Which specific DNA bases are targeted by phosphoramide mustard?
A2: Phosphoramide mustard preferentially alkylates guanine bases within DNA. [] Interestingly, the sequence context surrounding the guanine influences the likelihood of alkylation. For example, guanines flanked by cytosines are less susceptible to 4-OOH-CPA induced damage. []
Q3: Is DNA crosslinking the sole mechanism by which 4-OOH-CPA induces cell death?
A3: While DNA crosslinking by phosphoramide mustard is considered the primary cytotoxic mechanism, 4-OOH-CPA also induces apoptosis, a programmed cell death pathway. [, , , ] This process involves specific morphological and biochemical changes, including DNA fragmentation and activation of caspase enzymes. [, , , ]
Q4: How does the pH of the environment influence 4-OOH-CPA's conversion to active metabolites?
A5: The conversion of 4-OOH-CPA's primary metabolite, 4-hydroxycyclophosphamide, into phosphoramide mustard and acrolein is influenced by the presence of general base catalysts like phosphate and bicarbonate. [, ] This conversion is more efficient at higher pH and catalyst concentrations. [, ] This pH dependency has significant implications for 4-OOH-CPA's urotoxic potential, as the pH and composition of urine can affect acrolein-induced bladder toxicity. []
Q5: Can the intracellular concentration of specific molecules modulate 4-OOH-CPA's cytotoxic activity?
A6: Yes, the intracellular concentration of bifunctional catalysts, such as inorganic phosphates, organic phosphates, and certain enzymes, can influence the conversion of 4-hydroxycyclophosphamide to phosphoramide mustard and acrolein. [] This suggests that variations in these catalysts between tumor cells and normal cells could potentially contribute to 4-OOH-CPA's oncotoxic specificity. []
Q6: How does the tumor suppressor protein p53 influence the response of cells to 4-OOH-CPA?
A7: The presence of functional p53 appears to protect cells, particularly those in organogenesis-stage limbs, from 4-OOH-CPA induced damage. [] p53 plays a crucial role in cell cycle arrest and apoptosis, both of which are triggered in response to DNA damage caused by 4-OOH-CPA. [] In the absence of p53, cells may accumulate DNA damage, leading to increased cell death by necrosis and more severe developmental abnormalities. []
Q7: What is the role of cytochrome c in 4-OOH-CPA induced cell death?
A8: Studies have shown that 4-OOH-CPA, alongside other teratogens like heat shock, can trigger the release of cytochrome c from mitochondria in embryonic cells. [] This release precedes the activation of caspase enzymes, indicating that cytochrome c release is an upstream event in the apoptotic pathway induced by 4-OOH-CPA. []
Q8: Are there cell types that display resistance to the apoptotic effects of 4-OOH-CPA?
A9: Yes, certain cell types, such as heart cells in day 9 mouse embryos, demonstrate remarkable resistance to the cell death-inducing effects of 4-OOH-CPA. [] This resistance is linked to the absence of cytochrome c release from mitochondria, highlighting the critical role of this event in 4-OOH-CPA induced apoptosis. []
Q9: How is 4-hydroperoxycyclophosphamide used in the context of autologous bone marrow transplantation?
A10: 4-OOH-CPA is employed ex vivo to purge bone marrow grafts of contaminating tumor cells prior to transplantation. [, , , , , , , ] This approach aims to reduce the risk of disease relapse after transplantation. [, , , , , , , ]
Q10: What are the challenges associated with using 4-OOH-CPA for ex vivo purging?
A11: While effective in eliminating certain tumor cells, 4-OOH-CPA can also damage healthy hematopoietic stem cells in the bone marrow graft. [, , , ] This damage can lead to delayed or incomplete hematopoietic recovery after transplantation, increasing the risk of infections and bleeding complications. [, , , ]
Q11: Are there strategies to enhance the efficacy and safety of 4-OOH-CPA purging?
A12: Several approaches are being explored to improve 4-OOH-CPA purging. One strategy involves combining 4-OOH-CPA with other agents, such as etoposide or immunotoxins, to enhance tumor cell killing while minimizing damage to healthy stem cells. [, ] Another approach focuses on optimizing the incubation conditions during ex vivo treatment, including the concentration of red blood cells and nucleated bone marrow cells, which can influence 4-OOH-CPA activity. []
Q12: Can the effectiveness of 4-OOH-CPA purging be predicted?
A13: Researchers are investigating potential biomarkers and assays to predict the success of 4-OOH-CPA purging. For instance, the CFU-GM (colony-forming unit-granulocyte, macrophage) content of the purged bone marrow graft has been correlated with the time to hematologic recovery after transplantation. [] This suggests that CFU-GM content could serve as a potential predictor of hematopoietic reconstitution. []
Q13: How does the sensitivity of leukemic cells to 4-OOH-CPA vary?
A14: The sensitivity of leukemic cells to 4-OOH-CPA can differ significantly depending on factors like the leukemia subtype, stage of differentiation, and prior treatment history. [, , , ] This variability highlights the need for individualized treatment strategies and underscores the importance of developing reliable methods to assess tumor cell sensitivity before ex vivo purging. [, , , ]
Q14: What are the potential advantages of intraarterial administration of 4-OOH-CPA for treating brain tumors?
A15: Intraarterial (i.a.) administration of 4-OOH-CPA for brain tumors offers potential advantages over intravenous (i.v.) administration. [] I.a. delivery allows for higher drug concentrations to be achieved directly within the tumor, while minimizing systemic exposure and toxicity. [] This targeted approach has been shown to improve survival in animal models of human glioma. []
Q15: Are there any alternatives to 4-OOH-CPA for ex vivo purging or cancer treatment?
A16: Yes, researchers are continually exploring alternative agents and strategies for both ex vivo purging and cancer treatment. For purging, options include other chemotherapeutic drugs, immunotoxins, and photodynamic therapy. [, , ] In the broader context of cancer treatment, targeted therapies, immunotherapy, and novel drug delivery systems are actively being developed and tested.
Avertissement et informations sur les produits de recherche in vitro
Veuillez noter que tous les articles et informations sur les produits présentés sur BenchChem sont destinés uniquement à des fins informatives. Les produits disponibles à l'achat sur BenchChem sont spécifiquement conçus pour des études in vitro, qui sont réalisées en dehors des organismes vivants. Les études in vitro, dérivées du terme latin "in verre", impliquent des expériences réalisées dans des environnements de laboratoire contrôlés à l'aide de cellules ou de tissus. Il est important de noter que ces produits ne sont pas classés comme médicaments et n'ont pas reçu l'approbation de la FDA pour la prévention, le traitement ou la guérison de toute condition médicale, affection ou maladie. Nous devons souligner que toute forme d'introduction corporelle de ces produits chez les humains ou les animaux est strictement interdite par la loi. Il est essentiel de respecter ces directives pour assurer la conformité aux normes légales et éthiques en matière de recherche et d'expérimentation.