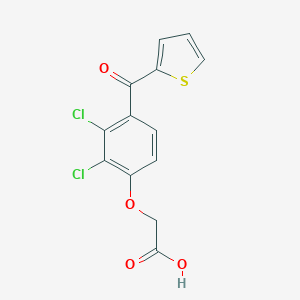
Tienilic acid
Vue d'ensemble
Description
Molecular Structure Analysis
Tienilic acid has a molecular formula of C13H8Cl2O4S . Its average mass is 331.171 Da and its monoisotopic mass is 329.952026 Da .
Physical And Chemical Properties Analysis
Tienilic acid has a density of 1.5±0.1 g/cm3, a boiling point of 534.6±50.0 °C at 760 mmHg, and a flash point of 277.1±30.1 °C . It has 4 H bond acceptors, 1 H bond donor, and 5 freely rotating bonds .
Applications De Recherche Scientifique
Immunological Response in Hepatotoxicity
Tienilic acid has been associated with hepatotoxicity, which may be immune-mediated. Research has investigated the sensitization to drug-altered liver cell antigens and autoantigens, suggesting that the drug reaction is linked to an immune attack on normal liver cell components .
Influence on Hyperbilirubinemia
Studies have shown that Tienilic acid can enhance hyperbilirubinemia in certain rat models by inducing hepatic Multidrug Resistance–Associated Protein 3 (Mrp3) and Heme Oxygenase-1 (Ho-1). This suggests a role for Tienilic acid in modulating bilirubin transport and formation in the liver .
Drug Metabolism and Enzyme Interaction
Tienilic acid is metabolized by the hepatic drug-metabolizing enzyme CYP2C9. It forms a reactive intermediate that covalently binds to macromolecules, including the metabolizing enzyme itself. This interaction is significant for understanding drug metabolism and potential drug-drug interactions .
Pharmacological Effects on Renal Function
As a diuretic, Tienilic acid was noted for its uric acid-lowering activity. Although withdrawn from the market due to hepatotoxicity, its pharmacological effects on renal function and uric acid levels remain of interest for research in related therapeutic areas .
Mechanistic Studies in Drug-Induced Liver Injury
Tienilic acid’s role in drug-induced liver injury (DILI) provides a model for studying the mechanisms of DILI, including the identification of biomarkers and the development of preventive strategies against such adverse drug reactions .
Exploration of Alternative Therapeutic Uses
Despite its hepatotoxicity, the biochemical pathways influenced by Tienilic acid offer a basis for exploring alternative therapeutic uses. Its effects on liver enzymes, transport proteins, and bilirubin metabolism could inform the development of new drugs with similar beneficial effects but reduced toxicity .
Safety and Hazards
Mécanisme D'action
Tienilic acid, also known as Ticrynafen, is a diuretic drug with uric acid-lowering action . It was formerly marketed for the treatment of hypertension . It was withdrawn in 1982 after case reports in the united states suggested a link between ticrynafen and hepatitis .
Target of Action
Tienilic acid acts as a suicide substrate at the cytochrome P450 enzymes involved in drug metabolism . The primary targets of Tienilic acid are the CYP2C9 enzymes . These enzymes play a crucial role in the metabolism of both endogenous substances and xenobiotics .
Mode of Action
The metabolic reaction carried out by these enzymes converts Tienilic acid to a thiophene sulfoxide , which is highly electrophilic . This encourages a Michael reaction leading to alkylation of a thiol group in the enzyme’s active site . Loss of water from the thiophene sulfoxide restores the thiophene ring and results in Tienilic acid being covalently linked to the enzyme, thus inhibiting the enzyme irreversibly .
Biochemical Pathways
The biochemical pathways affected by Tienilic acid are related to its interaction with the cytochrome P450 enzymes . The inhibition of these enzymes disrupts their normal metabolic functions, affecting the metabolism of various substances in the body .
Pharmacokinetics
Tienilic acid is extensively bound to plasma proteins (more than 98%) . The apparent volume of distribution of the drug is close to that of plasma volume . Tienilic acid disappears rapidly from the plasma according to a first-order kinetics, and the plasma half-life ranges from 1.6 to 2.4 hours . It is excreted by a saturable renal tubular transport mechanism .
Result of Action
The pharmacodynamic effects of Tienilic acid develop quickly, with the uricosuric effect being very impressive and the natriuretic effect moderate . These effects disappear in about 8 hours . An inverse relationship was found between the starting plasma uric acid level in an individual and the maximal uric acid clearance — the higher the plasma uric acid level, the lower was the maximum effect .
Propriétés
IUPAC Name |
2-[2,3-dichloro-4-(thiophene-2-carbonyl)phenoxy]acetic acid | |
---|---|---|
Source | PubChem | |
URL | https://pubchem.ncbi.nlm.nih.gov | |
Description | Data deposited in or computed by PubChem | |
InChI |
InChI=1S/C13H8Cl2O4S/c14-11-7(13(18)9-2-1-5-20-9)3-4-8(12(11)15)19-6-10(16)17/h1-5H,6H2,(H,16,17) | |
Source | PubChem | |
URL | https://pubchem.ncbi.nlm.nih.gov | |
Description | Data deposited in or computed by PubChem | |
InChI Key |
AGHANLSBXUWXTB-UHFFFAOYSA-N | |
Source | PubChem | |
URL | https://pubchem.ncbi.nlm.nih.gov | |
Description | Data deposited in or computed by PubChem | |
Canonical SMILES |
C1=CSC(=C1)C(=O)C2=C(C(=C(C=C2)OCC(=O)O)Cl)Cl | |
Source | PubChem | |
URL | https://pubchem.ncbi.nlm.nih.gov | |
Description | Data deposited in or computed by PubChem | |
Molecular Formula |
C13H8Cl2O4S | |
Source | PubChem | |
URL | https://pubchem.ncbi.nlm.nih.gov | |
Description | Data deposited in or computed by PubChem | |
DSSTOX Substance ID |
DTXSID4023670 | |
Record name | Ticrynafen | |
Source | EPA DSSTox | |
URL | https://comptox.epa.gov/dashboard/DTXSID4023670 | |
Description | DSSTox provides a high quality public chemistry resource for supporting improved predictive toxicology. | |
Molecular Weight |
331.2 g/mol | |
Source | PubChem | |
URL | https://pubchem.ncbi.nlm.nih.gov | |
Description | Data deposited in or computed by PubChem | |
Product Name |
Tienilic acid | |
CAS RN |
40180-04-9 | |
Record name | Tienilic acid | |
Source | CAS Common Chemistry | |
URL | https://commonchemistry.cas.org/detail?cas_rn=40180-04-9 | |
Description | CAS Common Chemistry is an open community resource for accessing chemical information. Nearly 500,000 chemical substances from CAS REGISTRY cover areas of community interest, including common and frequently regulated chemicals, and those relevant to high school and undergraduate chemistry classes. This chemical information, curated by our expert scientists, is provided in alignment with our mission as a division of the American Chemical Society. | |
Explanation | The data from CAS Common Chemistry is provided under a CC-BY-NC 4.0 license, unless otherwise stated. | |
Record name | Ticrynafen [USAN] | |
Source | ChemIDplus | |
URL | https://pubchem.ncbi.nlm.nih.gov/substance/?source=chemidplus&sourceid=0040180049 | |
Description | ChemIDplus is a free, web search system that provides access to the structure and nomenclature authority files used for the identification of chemical substances cited in National Library of Medicine (NLM) databases, including the TOXNET system. | |
Record name | Tienilic acid | |
Source | DrugBank | |
URL | https://www.drugbank.ca/drugs/DB04831 | |
Description | The DrugBank database is a unique bioinformatics and cheminformatics resource that combines detailed drug (i.e. chemical, pharmacological and pharmaceutical) data with comprehensive drug target (i.e. sequence, structure, and pathway) information. | |
Explanation | Creative Common's Attribution-NonCommercial 4.0 International License (http://creativecommons.org/licenses/by-nc/4.0/legalcode) | |
Record name | Ticrynafen | |
Source | EPA DSSTox | |
URL | https://comptox.epa.gov/dashboard/DTXSID4023670 | |
Description | DSSTox provides a high quality public chemistry resource for supporting improved predictive toxicology. | |
Record name | Tienilic acid | |
Source | European Chemicals Agency (ECHA) | |
URL | https://echa.europa.eu/substance-information/-/substanceinfo/100.049.825 | |
Description | The European Chemicals Agency (ECHA) is an agency of the European Union which is the driving force among regulatory authorities in implementing the EU's groundbreaking chemicals legislation for the benefit of human health and the environment as well as for innovation and competitiveness. | |
Explanation | Use of the information, documents and data from the ECHA website is subject to the terms and conditions of this Legal Notice, and subject to other binding limitations provided for under applicable law, the information, documents and data made available on the ECHA website may be reproduced, distributed and/or used, totally or in part, for non-commercial purposes provided that ECHA is acknowledged as the source: "Source: European Chemicals Agency, http://echa.europa.eu/". Such acknowledgement must be included in each copy of the material. ECHA permits and encourages organisations and individuals to create links to the ECHA website under the following cumulative conditions: Links can only be made to webpages that provide a link to the Legal Notice page. | |
Record name | TICRYNAFEN | |
Source | FDA Global Substance Registration System (GSRS) | |
URL | https://gsrs.ncats.nih.gov/ginas/app/beta/substances/HC95205SY4 | |
Description | The FDA Global Substance Registration System (GSRS) enables the efficient and accurate exchange of information on what substances are in regulated products. Instead of relying on names, which vary across regulatory domains, countries, and regions, the GSRS knowledge base makes it possible for substances to be defined by standardized, scientific descriptions. | |
Explanation | Unless otherwise noted, the contents of the FDA website (www.fda.gov), both text and graphics, are not copyrighted. They are in the public domain and may be republished, reprinted and otherwise used freely by anyone without the need to obtain permission from FDA. Credit to the U.S. Food and Drug Administration as the source is appreciated but not required. | |
Retrosynthesis Analysis
AI-Powered Synthesis Planning: Our tool employs the Template_relevance Pistachio, Template_relevance Bkms_metabolic, Template_relevance Pistachio_ringbreaker, Template_relevance Reaxys, Template_relevance Reaxys_biocatalysis model, leveraging a vast database of chemical reactions to predict feasible synthetic routes.
One-Step Synthesis Focus: Specifically designed for one-step synthesis, it provides concise and direct routes for your target compounds, streamlining the synthesis process.
Accurate Predictions: Utilizing the extensive PISTACHIO, BKMS_METABOLIC, PISTACHIO_RINGBREAKER, REAXYS, REAXYS_BIOCATALYSIS database, our tool offers high-accuracy predictions, reflecting the latest in chemical research and data.
Strategy Settings
Precursor scoring | Relevance Heuristic |
---|---|
Min. plausibility | 0.01 |
Model | Template_relevance |
Template Set | Pistachio/Bkms_metabolic/Pistachio_ringbreaker/Reaxys/Reaxys_biocatalysis |
Top-N result to add to graph | 6 |
Feasible Synthetic Routes
Q & A
Q1: What is the primary mechanism of action of tienilic acid?
A1: Tienilic acid primarily acts as a diuretic by inhibiting the reabsorption of sodium and uric acid in the cortical diluting segment of the distal nephron within the kidney. [] This inhibition leads to increased excretion of sodium, water, and uric acid in the urine, resulting in its diuretic and uricosuric effects. [, ]
Q2: How does tienilic acid's uricosuric effect differ from that of probenecid?
A2: While both tienilic acid and probenecid exhibit uricosuric effects, their mechanisms differ. Tienilic acid primarily inhibits urate reabsorption, while probenecid inhibits both urate reabsorption and secretion, ultimately leading to a greater increase in urinary supersaturation with uric acid and monosodium urate compared to tienilic acid. [, ]
Q3: Does tienilic acid affect renal handling of other electrolytes like potassium?
A3: Yes, tienilic acid can cause mild hypokalaemia, similar to other thiazide diuretics. [, , , , , , ] This effect is attributed to increased potassium excretion in the urine due to enhanced sodium delivery to the distal nephron. [] Co-administration with potassium-sparing diuretics or potassium supplements can mitigate this effect. [, , ]
Q4: What is the role of cytochrome P450 2C9 (CYP2C9) in tienilic acid metabolism?
A4: CYP2C9 is the primary enzyme responsible for the 5-hydroxylation of tienilic acid in the liver. [, , , ] This metabolic pathway is significant because it leads to the formation of reactive metabolites that can covalently bind to CYP2C9, leading to its inactivation. [, ]
Q5: How does tienilic acid's interaction with CYP2C9 relate to its hepatotoxicity?
A5: The reactive metabolites generated during tienilic acid metabolism by CYP2C9 are thought to play a key role in its hepatotoxicity. [, , , ] These metabolites can covalently bind to CYP2C9 and other proteins, potentially triggering an immune response and leading to hepatitis in susceptible individuals. [, , , ]
Q6: What is the molecular formula and weight of tienilic acid?
A6: The molecular formula of tienilic acid is C13H8Cl2O4S, and its molecular weight is 331.18 g/mol.
Q7: Is there any spectroscopic data available for tienilic acid?
A7: While specific spectroscopic data is not detailed in the provided research, various spectroscopic techniques were likely employed to characterize tienilic acid and its derivatives. These may include techniques like nuclear magnetic resonance (NMR) spectroscopy, infrared (IR) spectroscopy, and mass spectrometry (MS). []
Q8: How do structural modifications of tienilic acid affect its interaction with CYP2C9?
A8: Research suggests that the presence of an acidic function, such as a carboxylic acid, phenol, or tetrazole, is crucial for tienilic acid's recognition and metabolism by CYP2C9. [] Replacing this acidic function with other moieties significantly reduces or eliminates its binding affinity and metabolism by CYP2C9. []
Q9: How is tienilic acid absorbed and distributed in the body?
A9: Tienilic acid is well-absorbed following oral administration and is extensively bound to plasma proteins, primarily albumin. [] This high protein binding results in lower tissue concentrations compared to plasma concentrations. []
Q10: How is tienilic acid eliminated from the body?
A10: Tienilic acid is rapidly cleared from the body through both metabolism and renal excretion. [] Approximately 30% of the administered dose is excreted unchanged in the urine, while the remaining portion is metabolized, primarily by CYP2C9 in the liver. [, ]
Q11: Does gender affect the pharmacokinetics of tienilic acid?
A11: Yes, there are gender differences in the renal excretion of tienilic acid, with males excreting the drug and its metabolites more efficiently than females. [] This difference in excretion may lead to variations in drug exposure and potentially influence the risk of adverse effects between genders.
Q12: What is the typical dose range of tienilic acid used in clinical trials for hypertension?
A12: Clinical trials evaluating the antihypertensive effect of tienilic acid commonly used a dose range of 250 mg to 500 mg per day. [, , , , , , , , , ]
Q13: What were the main reasons for withdrawing tienilic acid from the market?
A13: Tienilic acid was withdrawn from the market due to a low incidence of severe hepatotoxicity, including cases of fatal hepatic failure, observed in some patients. [, , , , ]
Q14: Were there any other notable adverse effects associated with tienilic acid?
A14: Besides hepatotoxicity, tienilic acid could also cause mild hypokalaemia, similar to other thiazide diuretics. [, , , , , , ] Acute gouty attacks were also reported, particularly at the beginning of treatment. [, ]
Q15: How does tienilic acid induce an immune response?
A15: Tienilic acid-induced hepatitis is classified as an autoimmune hepatitis characterized by the presence of anti-liver and -kidney microsomal type 2 (anti-LKM2) autoantibodies in the serum of affected individuals. [, , , ] These antibodies are specifically directed against CYP2C9, the enzyme involved in tienilic acid metabolism. [, , ]
Q16: What is the significance of anti-LKM2 antibodies in the context of tienilic acid-induced hepatitis?
A16: The presence of anti-LKM2 antibodies is considered a specific marker for tienilic acid-induced hepatitis. [, , , ] These antibodies are rarely found in other liver diseases or in individuals exposed to tienilic acid without developing hepatitis. [, ]
Q17: What is the epitope recognized by anti-LKM2 antibodies on CYP2C9?
A17: Research suggests that anti-LKM2 antibodies recognize a complex, conformational epitope on CYP2C9 formed by the interaction of three distinct regions: amino acids 314-322, 345-356, and 439-455. []
Q18: What analytical techniques were used to study the metabolism of tienilic acid?
A18: Various analytical techniques were employed to study tienilic acid metabolism, including high-performance liquid chromatography (HPLC) coupled with electrospray ionization mass spectrometry (ESI-MS). [] This technique enabled the identification and characterization of tienilic acid adducts formed with CYP2C9 during its metabolism. []
Q19: How was the covalent binding of tienilic acid metabolites to proteins assessed?
A19: The covalent binding of tienilic acid metabolites to microsomal proteins was assessed by incubating liver microsomes with radiolabeled tienilic acid. [] Following incubation, unbound tienilic acid and its metabolites were removed, and the amount of radioactivity associated with the protein fraction was measured. []
Avertissement et informations sur les produits de recherche in vitro
Veuillez noter que tous les articles et informations sur les produits présentés sur BenchChem sont destinés uniquement à des fins informatives. Les produits disponibles à l'achat sur BenchChem sont spécifiquement conçus pour des études in vitro, qui sont réalisées en dehors des organismes vivants. Les études in vitro, dérivées du terme latin "in verre", impliquent des expériences réalisées dans des environnements de laboratoire contrôlés à l'aide de cellules ou de tissus. Il est important de noter que ces produits ne sont pas classés comme médicaments et n'ont pas reçu l'approbation de la FDA pour la prévention, le traitement ou la guérison de toute condition médicale, affection ou maladie. Nous devons souligner que toute forme d'introduction corporelle de ces produits chez les humains ou les animaux est strictement interdite par la loi. Il est essentiel de respecter ces directives pour assurer la conformité aux normes légales et éthiques en matière de recherche et d'expérimentation.