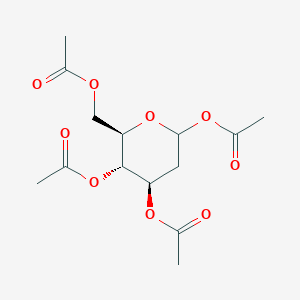
1,3,4,6-Tetra-O-acetyl-2-deoxy-D-glucopyranose
Vue d'ensemble
Description
1,3,4,6-Tetra-O-acetyl-2-deoxy-D-glucopyranose: is a derivative of glucose, specifically a tetraacetylated form of 2-deoxy-D-glucose. This compound is often used in organic synthesis and biochemical research due to its unique structural properties and reactivity.
Méthodes De Préparation
Synthetic Routes and Reaction Conditions: 1,3,4,6-Tetra-O-acetyl-2-deoxy-D-glucopyranose can be synthesized through the acetylation of 2-deoxy-D-glucose. The process typically involves the use of acetic anhydride and a catalyst such as pyridine. The reaction is carried out under anhydrous conditions to prevent hydrolysis of the acetyl groups.
Industrial Production Methods: In an industrial setting, the synthesis of this compound follows similar principles but on a larger scale. The reaction conditions are optimized for higher yields and purity, often involving continuous flow reactors and automated systems to control temperature and reagent addition.
Analyse Des Réactions Chimiques
Types of Reactions: 1,3,4,6-Tetra-O-acetyl-2-deoxy-D-glucopyranose undergoes various chemical reactions, including:
Hydrolysis: The acetyl groups can be hydrolyzed under acidic or basic conditions to yield 2-deoxy-D-glucose.
Substitution: The acetyl groups can be substituted with other functional groups using appropriate reagents.
Oxidation: The compound can be oxidized to form corresponding carboxylic acids or aldehydes.
Common Reagents and Conditions:
Hydrolysis: Acidic (e.g., hydrochloric acid) or basic (e.g., sodium hydroxide) conditions.
Substitution: Reagents such as sodium methoxide or other nucleophiles.
Oxidation: Oxidizing agents like potassium permanganate or chromium trioxide.
Major Products:
Hydrolysis: 2-deoxy-D-glucose.
Substitution: Various substituted derivatives depending on the nucleophile used.
Oxidation: Carboxylic acids or aldehydes.
Applications De Recherche Scientifique
1,3,4,6-Tetra-O-acetyl-2-deoxy-D-glucopyranose has several applications in scientific research:
Chemistry: Used as a building block in the synthesis of more complex molecules.
Biology: Employed in studies of carbohydrate metabolism and enzyme activity.
Medicine: Investigated for its potential in drug development, particularly in targeting metabolic pathways.
Industry: Utilized in the production of specialty chemicals and as an intermediate in various synthetic processes.
Mécanisme D'action
The mechanism of action of 1,3,4,6-Tetra-O-acetyl-2-deoxy-D-glucopyranose involves its interaction with enzymes and metabolic pathways. The acetyl groups can be cleaved by esterases, releasing 2-deoxy-D-glucose, which can then participate in glycolysis and other metabolic processes. The compound’s acetylated form can also interact with specific proteins and enzymes, influencing their activity and function.
Comparaison Avec Des Composés Similaires
1,3,4,6-Tetra-O-acetyl-2-amino-2-deoxy-D-glucopyranose: Similar structure but with an amino group instead of a hydroxyl group.
2-Acetamido-2-deoxy-D-glucopyranose 1,3,4,6-tetraacetate: Another acetylated derivative with an acetamido group.
1,3,4,6-Tetra-O-acetyl-D-glucopyranose: Similar but without the deoxy modification.
Uniqueness: 1,3,4,6-Tetra-O-acetyl-2-deoxy-D-glucopyranose is unique due to the absence of a hydroxyl group at the second carbon position, which significantly alters its reactivity and interaction with biological molecules compared to its hydroxylated counterparts.
Activité Biologique
1,3,4,6-Tetra-O-acetyl-2-deoxy-D-glucopyranose (TDA) is a derivative of 2-deoxy-D-glucose (2-DG), which has gained attention for its potential biological activities, particularly in the context of viral infections and metabolic disorders. This article reviews the biological activity of TDA, focusing on its mechanisms of action, effects on cellular metabolism, and implications for therapeutic applications.
Chemical Structure and Properties
This compound is characterized by the presence of acetyl groups at positions 1, 3, 4, and 6 on the glucopyranose ring. This structural modification enhances its solubility and biological activity compared to its parent compound, D-glucose.
Property | Value |
---|---|
Molecular Formula | C₁₆H₂₃NO₁₀ |
Molecular Weight | 389.37 g/mol |
Melting Point | 184-189 °C |
CAS Number | 7772-79-4 |
Mechanisms of Biological Activity
TDA exhibits several biological activities primarily through its interference with cellular glucose metabolism and glycosylation processes:
- Inhibition of Glycolysis : TDA acts as a glucose antimetabolite by inhibiting glycolytic enzymes such as hexokinase. This inhibition leads to reduced ATP production in cells, which is particularly detrimental to rapidly dividing cells like those in tumors or virally infected tissues .
- Impact on Glycosylation : By mimicking glucose, TDA disrupts the normal glycosylation processes essential for viral replication and cell signaling. This disruption can lead to endoplasmic reticulum stress and activation of the unfolded protein response (UPR), further impairing cellular function .
- Antiviral Properties : In studies involving SARS-CoV-2, TDA demonstrated superior binding energies compared to other derivatives of 2-DG, suggesting enhanced efficacy in inhibiting viral proliferation by limiting the energy supply necessary for viral replication .
Research Findings and Case Studies
Several studies have evaluated the biological effects of TDA:
- Reduction of Glucosamine Incorporation : A study revealed that TDA significantly reduced the incorporation of D-[3H]glucosamine into glycosaminoglycans (GAGs) without affecting total protein synthesis. This suggests that TDA competes with glucosamine in metabolic pathways critical for GAG synthesis .
- Cell Culture Studies : In primary hepatocyte cultures, TDA was shown to inhibit D-[3H]glucosamine uptake effectively. The concentration-dependent reduction indicates a potential role in modulating metabolic pathways involved in GAG production .
Potential Applications
The unique properties of TDA suggest several potential applications:
- Cancer Therapy : Given its ability to inhibit glycolysis and glycosylation, TDA may serve as a therapeutic agent in cancer treatment by starving tumor cells of energy and disrupting their growth signals.
- Viral Infections : The antiviral properties observed in laboratory settings indicate that TDA could be developed as a treatment option for viral infections, including COVID-19 .
Propriétés
IUPAC Name |
[(2R,3S,4R)-3,4,6-triacetyloxyoxan-2-yl]methyl acetate | |
---|---|---|
Source | PubChem | |
URL | https://pubchem.ncbi.nlm.nih.gov | |
Description | Data deposited in or computed by PubChem | |
InChI |
InChI=1S/C14H20O9/c1-7(15)19-6-12-14(22-10(4)18)11(20-8(2)16)5-13(23-12)21-9(3)17/h11-14H,5-6H2,1-4H3/t11-,12-,13?,14+/m1/s1 | |
Source | PubChem | |
URL | https://pubchem.ncbi.nlm.nih.gov | |
Description | Data deposited in or computed by PubChem | |
InChI Key |
KLEORKVJPIJWNG-VMXNZORSSA-N | |
Source | PubChem | |
URL | https://pubchem.ncbi.nlm.nih.gov | |
Description | Data deposited in or computed by PubChem | |
Canonical SMILES |
CC(=O)OCC1C(C(CC(O1)OC(=O)C)OC(=O)C)OC(=O)C | |
Source | PubChem | |
URL | https://pubchem.ncbi.nlm.nih.gov | |
Description | Data deposited in or computed by PubChem | |
Isomeric SMILES |
CC(=O)OC[C@@H]1[C@H]([C@@H](CC(O1)OC(=O)C)OC(=O)C)OC(=O)C | |
Source | PubChem | |
URL | https://pubchem.ncbi.nlm.nih.gov | |
Description | Data deposited in or computed by PubChem | |
Molecular Formula |
C14H20O9 | |
Source | PubChem | |
URL | https://pubchem.ncbi.nlm.nih.gov | |
Description | Data deposited in or computed by PubChem | |
DSSTOX Substance ID |
DTXSID50447840 | |
Record name | 1,3,4,6-Tetra-O-acetyl-2-deoxy-D-glucopyranose | |
Source | EPA DSSTox | |
URL | https://comptox.epa.gov/dashboard/DTXSID50447840 | |
Description | DSSTox provides a high quality public chemistry resource for supporting improved predictive toxicology. | |
Molecular Weight |
332.30 g/mol | |
Source | PubChem | |
URL | https://pubchem.ncbi.nlm.nih.gov | |
Description | Data deposited in or computed by PubChem | |
CAS No. |
69515-91-9 | |
Record name | 1,3,4,6-Tetra-O-acetyl-2-deoxy-D-glucopyranose | |
Source | EPA DSSTox | |
URL | https://comptox.epa.gov/dashboard/DTXSID50447840 | |
Description | DSSTox provides a high quality public chemistry resource for supporting improved predictive toxicology. | |
Retrosynthesis Analysis
AI-Powered Synthesis Planning: Our tool employs the Template_relevance Pistachio, Template_relevance Bkms_metabolic, Template_relevance Pistachio_ringbreaker, Template_relevance Reaxys, Template_relevance Reaxys_biocatalysis model, leveraging a vast database of chemical reactions to predict feasible synthetic routes.
One-Step Synthesis Focus: Specifically designed for one-step synthesis, it provides concise and direct routes for your target compounds, streamlining the synthesis process.
Accurate Predictions: Utilizing the extensive PISTACHIO, BKMS_METABOLIC, PISTACHIO_RINGBREAKER, REAXYS, REAXYS_BIOCATALYSIS database, our tool offers high-accuracy predictions, reflecting the latest in chemical research and data.
Strategy Settings
Precursor scoring | Relevance Heuristic |
---|---|
Min. plausibility | 0.01 |
Model | Template_relevance |
Template Set | Pistachio/Bkms_metabolic/Pistachio_ringbreaker/Reaxys/Reaxys_biocatalysis |
Top-N result to add to graph | 6 |
Feasible Synthetic Routes
Q1: What is the significance of incorporating 1,3,4,6-Tetra-O-acetyl-2-deoxy-D-glucopyranose into the structure of nalidixic acid?
A1: The research aimed to enhance the antimicrobial properties of nalidixic acid while potentially improving its safety profile []. By conjugating nalidixic acid with D-(+)-glucosamine, the researchers synthesized novel compounds, including one featuring this compound []. This modification led to increased antimicrobial activity against several bacterial strains, including resistant ones like Methicillin-resistant Staphylococcus aureus (MRSA) and resistant Escherichia coli []. Notably, the modified compounds displayed lower cytotoxicity compared to the original nalidixic acid, suggesting a potential improvement in safety [].
Q2: How does the structure of the this compound-containing compound relate to its antimicrobial activity?
A2: While the exact mechanism of action wasn't fully elucidated in the research, the study suggests that the inclusion of the glucosamine moiety, particularly in the form of this compound, contributes significantly to the enhanced antimicrobial activity []. This could be due to improved interaction with bacterial targets, altered uptake mechanisms, or a combination of factors. Further research, including investigations into structure-activity relationships, is needed to understand the precise mechanisms underlying the observed antimicrobial effects [].
Avertissement et informations sur les produits de recherche in vitro
Veuillez noter que tous les articles et informations sur les produits présentés sur BenchChem sont destinés uniquement à des fins informatives. Les produits disponibles à l'achat sur BenchChem sont spécifiquement conçus pour des études in vitro, qui sont réalisées en dehors des organismes vivants. Les études in vitro, dérivées du terme latin "in verre", impliquent des expériences réalisées dans des environnements de laboratoire contrôlés à l'aide de cellules ou de tissus. Il est important de noter que ces produits ne sont pas classés comme médicaments et n'ont pas reçu l'approbation de la FDA pour la prévention, le traitement ou la guérison de toute condition médicale, affection ou maladie. Nous devons souligner que toute forme d'introduction corporelle de ces produits chez les humains ou les animaux est strictement interdite par la loi. Il est essentiel de respecter ces directives pour assurer la conformité aux normes légales et éthiques en matière de recherche et d'expérimentation.