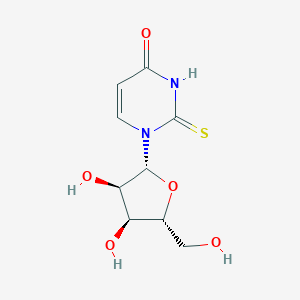
2-Thiouridine
Vue d'ensemble
Description
2-Thiouridine is a thiouridine in which the oxygen replaced by sulfur is that at C-2. It is a thiouridine and a nucleoside analogue . It is a metabolite found in or produced by Escherichia coli (strain K12, MG1655) . It has been identified as a broad-spectrum antiviral nucleoside analogue against positive-strand RNA viruses .
Synthesis Analysis
A gene (ttuC (two-thiouridine C), a thiF / moeB homologue) responsible for the synthesis of s2T in T. thermophilus has been identified, and it has been demonstrated that thiocarboxylated TtuB functions as a sulfur donor for s2T synthesis in vitro .
Molecular Structure Analysis
2-Thiouridine is a modified nucleobase found in tRNAs that is known to stabilize U:A base pairs and modestly destabilize U:G wobble pairs . The recently reported crystal structures of s2U-containing RNA duplexes do not .
Chemical Reactions Analysis
The main hurdles for mRNA clinical translation are the stability, translation efficiency, and immunostimulatory potential of exogenous mRNA . Current mRNA synthesis methods fail to remove impurities such as double-stranded RNA and RNA fragments, which reduce the therapeutic efficacy and cause undesired biological responses in clinical use . Replacing partial nucleosides with 2-thiouridine and 5-methylcytidine reduces RIG-1 activation .
Physical And Chemical Properties Analysis
2-Thiouridine is a modified base found in the tRNA of certain extreme thermophiles. 2-Thiouridine may be used to study the physical chemical properties that confer high stability to tRNA and other nucleic acid structures .
Applications De Recherche Scientifique
Broad-Spectrum Antiviral Agent
s2U has been identified as a broad-spectrum antiviral nucleoside analogue . It exhibits antiviral activity against SARS-CoV-2 and its variants of concern, including the Delta and Omicron variants . This makes it a potential therapeutic agent in the fight against COVID-19.
Inhibitor of RNA Synthesis
s2U inhibits RNA synthesis catalyzed by viral RNA-dependent RNA polymerase . This reduces viral RNA replication, which has been shown to improve the survival rate of mice infected with SARS-CoV-2 .
Effective Against Dengue Virus (DENV)
In addition to SARS-CoV-2, s2U also exhibits antiviral activity against a number of other positive-sense single-stranded RNA (ssRNA+) viruses, including DENV . This broad-spectrum antiviral activity makes s2U a potential candidate for treating various viral infections.
Impact on tRNA Modifications
s2U plays a crucial role in posttranscriptional modifications to tRNA . These modifications are critical elements for the folding and functionality of tRNA molecules .
Indicator of Sulfur Availability
The relative abundance of 2-thiouridine-modified tRNA (s2U) responds to sulfur availability in the growth medium in a dose-dependent manner . This makes s2U a potential marker for sulfur availability in certain organisms .
Inhibitor of Murine Norovirus-1 (MNV-1) Replication
s2U has been reported to inhibit the replication of murine norovirus-1 (MNV-1), which belongs to the Caliciviridae family . This further extends the range of viruses against which s2U exhibits antiviral activity.
Safety and Hazards
Avoid dust formation. Avoid breathing mist, gas, or vapors. Avoid contacting with skin and eye. Use personal protective equipment. Wear chemical impermeable gloves. Ensure adequate ventilation. Remove all sources of ignition. Evacuate personnel to safe areas. Keep people away from and upwind of spill/leak .
Orientations Futures
The presence of sulfur in cofactors has been appreciated for over a century, but the trafficking and delivery of sulfur to cofactors and nucleosides is still not fully understood . Mechanisms for the use of persulfide groups are illustrated with the relatively simple case of 4-thiourdine generation, and further possibilities are illuminated by the 2-thiouridine and cofactor biosynthetic systems .
Mécanisme D'action
Target of Action
2-Thiouridine (s2U) is a broad-spectrum antiviral nucleoside analogue that targets positive-sense single-stranded RNA (ssRNA+) viruses . These viruses include SARS-CoV-2 and its variants of concern, such as the Delta and Omicron variants, as well as other viruses like Dengue virus (DENV) . The primary target of s2U is the viral RNA-dependent RNA polymerase (RdRp), an enzyme that catalyzes RNA synthesis .
Mode of Action
The mode of action of s2U involves inhibiting the RNA synthesis catalyzed by the viral RdRp . By doing so, it reduces viral RNA replication, thereby limiting the ability of the virus to multiply and spread .
Biochemical Pathways
The biochemical pathways affected by s2U involve the sulfur metabolism. Sulfur availability impacts the accumulation of the 2-thiouridine tRNA modification in certain organisms . The enzymes involved in this modification are essential, confirming the well-established role of s2U in maintaining translational efficiency .
Pharmacokinetics
In pharmacokinetic analysis, it was found that once-daily intravenous and twice-daily oral administration of s2U maintains the validated concentration of more than 50% effective concentration (EC 50) or 90% effective concentration (EC 90) against DENV2 and SARS-CoV-2 . This suggests that s2U has good bioavailability and can reach therapeutic concentrations in the body with regular dosing.
Result of Action
The result of s2U’s action is the improved survival rate of mice infected with SARS-CoV-2 or DENV in animal models .
Action Environment
The action of s2U may be influenced by environmental factors such as sulfur availability . .
Propriétés
IUPAC Name |
1-[(2R,3R,4S,5R)-3,4-dihydroxy-5-(hydroxymethyl)oxolan-2-yl]-2-sulfanylidenepyrimidin-4-one | |
---|---|---|
Source | PubChem | |
URL | https://pubchem.ncbi.nlm.nih.gov | |
Description | Data deposited in or computed by PubChem | |
InChI |
InChI=1S/C9H12N2O5S/c12-3-4-6(14)7(15)8(16-4)11-2-1-5(13)10-9(11)17/h1-2,4,6-8,12,14-15H,3H2,(H,10,13,17)/t4-,6-,7-,8-/m1/s1 | |
Source | PubChem | |
URL | https://pubchem.ncbi.nlm.nih.gov | |
Description | Data deposited in or computed by PubChem | |
InChI Key |
GJTBSTBJLVYKAU-XVFCMESISA-N | |
Source | PubChem | |
URL | https://pubchem.ncbi.nlm.nih.gov | |
Description | Data deposited in or computed by PubChem | |
Canonical SMILES |
C1=CN(C(=S)NC1=O)C2C(C(C(O2)CO)O)O | |
Source | PubChem | |
URL | https://pubchem.ncbi.nlm.nih.gov | |
Description | Data deposited in or computed by PubChem | |
Isomeric SMILES |
C1=CN(C(=S)NC1=O)[C@H]2[C@@H]([C@@H]([C@H](O2)CO)O)O | |
Source | PubChem | |
URL | https://pubchem.ncbi.nlm.nih.gov | |
Description | Data deposited in or computed by PubChem | |
Molecular Formula |
C9H12N2O5S | |
Source | PubChem | |
URL | https://pubchem.ncbi.nlm.nih.gov | |
Description | Data deposited in or computed by PubChem | |
DSSTOX Substance ID |
DTXSID90174087 | |
Record name | 2-Thiouridine | |
Source | EPA DSSTox | |
URL | https://comptox.epa.gov/dashboard/DTXSID90174087 | |
Description | DSSTox provides a high quality public chemistry resource for supporting improved predictive toxicology. | |
Molecular Weight |
260.27 g/mol | |
Source | PubChem | |
URL | https://pubchem.ncbi.nlm.nih.gov | |
Description | Data deposited in or computed by PubChem | |
Product Name |
2-Thiouridine | |
CAS RN |
20235-78-3 | |
Record name | 2-Thiouridine | |
Source | ChemIDplus | |
URL | https://pubchem.ncbi.nlm.nih.gov/substance/?source=chemidplus&sourceid=0020235783 | |
Description | ChemIDplus is a free, web search system that provides access to the structure and nomenclature authority files used for the identification of chemical substances cited in National Library of Medicine (NLM) databases, including the TOXNET system. | |
Record name | 2-Thiouridine | |
Source | EPA DSSTox | |
URL | https://comptox.epa.gov/dashboard/DTXSID90174087 | |
Description | DSSTox provides a high quality public chemistry resource for supporting improved predictive toxicology. | |
Retrosynthesis Analysis
AI-Powered Synthesis Planning: Our tool employs the Template_relevance Pistachio, Template_relevance Bkms_metabolic, Template_relevance Pistachio_ringbreaker, Template_relevance Reaxys, Template_relevance Reaxys_biocatalysis model, leveraging a vast database of chemical reactions to predict feasible synthetic routes.
One-Step Synthesis Focus: Specifically designed for one-step synthesis, it provides concise and direct routes for your target compounds, streamlining the synthesis process.
Accurate Predictions: Utilizing the extensive PISTACHIO, BKMS_METABOLIC, PISTACHIO_RINGBREAKER, REAXYS, REAXYS_BIOCATALYSIS database, our tool offers high-accuracy predictions, reflecting the latest in chemical research and data.
Strategy Settings
Precursor scoring | Relevance Heuristic |
---|---|
Min. plausibility | 0.01 |
Model | Template_relevance |
Template Set | Pistachio/Bkms_metabolic/Pistachio_ringbreaker/Reaxys/Reaxys_biocatalysis |
Top-N result to add to graph | 6 |
Feasible Synthetic Routes
Q & A
Q1: How does the presence of 2-thiouridine in tRNA impact codon recognition?
A1: 2-thiouridine (s2U), often found at the wobble position (position 34) of the anticodon loop in tRNA, plays a crucial role in accurate codon recognition. The 2-thio group on the uridine base increases its conformational rigidity by favoring the C3'-endo ribose puckering. This restricted conformation ensures stable and accurate codon-anticodon pairing, specifically promoting the recognition of A over G at the 3' end of mRNA codons. [, , , , , ]
Q2: How does the 5-substituent on 2-thiouridine influence its interaction with guanosine in mRNA?
A2: The 5-substituent on 2-thiouridine significantly impacts its interaction with guanosine. tRNAs with 2-thiouridines containing aminoalkyl 5-substituents are partially ionized at physiological pH, favoring a zwitterionic form that interacts with guanosine through a “new wobble” pattern. In contrast, 2-thiouridines with non-ionizable 5-substituents preferentially bind to guanosine in a C-G-like Watson-Crick mode. []
Q3: What are the implications of 2-thiouridine deficiency on bacterial growth?
A3: Studies in Salmonella enterica demonstrate that the absence of both the 2-thio group and the 5-substituent ((c)mnm5) in tRNA results in non-viability across different temperatures. Mutants lacking either the 2-thio or the (c)mnm5 group exhibit poor growth, particularly at low temperatures. These findings suggest that 2-thiouridine plays a crucial role in maintaining translational fidelity and bacterial viability. []
Q4: What is the molecular formula and weight of 2-thiouridine?
A4: The molecular formula of 2-thiouridine is C9H12N2O5S, and its molecular weight is 260.27 g/mol.
Q5: How does the UV spectrum of 2-thiouridine differ from that of uridine?
A5: The presence of the sulfur atom at position 2 in 2-thiouridine results in a bathochromic shift in its UV spectrum compared to uridine. This shift is attributed to the increased electron delocalization over the pyrimidine ring due to the sulfur atom.
Q6: What is the role of the iron-sulfur cluster in the activity of the 2-thiouridine synthetase TtuA?
A7: The 2-thiouridine synthetase TtuA requires an oxygen-labile [4Fe-4S] cluster for its enzymatic activity. This cluster is coordinated by three conserved cysteine residues, with one iron atom exposed to the active site. The [4Fe-4S] cluster is essential for TtuA to accept sulfur from the sulfur donor protein TtuB and subsequently transfer it to tRNA for 2-thiouridine formation. []
Q7: How do molecular dynamics simulations contribute to our understanding of 2-thiouridine’s influence on RNA structure?
A8: Molecular dynamics simulations employing revised force field parameters for 2-thiouridine have demonstrated its significant impact on RNA structure. Simulations of a pentanucleotide sequence containing 2-thiouridine showed a conformational ensemble closer to the A-form helix observed in the crystal structure of HIV reverse-transcription primer tRNALys compared to the unmodified sequence. These findings highlight the role of 2-thiouridine in promoting a more ordered A-RNA-like structure. []
Q8: What insights have theoretical calculations provided into the conformational preferences of ribose in 2-thiouridine?
A9: DFT and MP2 calculations have revealed that the increased polarity of the C2-S2 bond in 2-thiouridine, influenced by the orientation of the 2'-OH group, leads to differences in bond lengths, atomic charges, and vibrational frequencies among different ribose conformers. The C3'-endo conformation of ribose, predominantly observed in 2-thiouridine, exhibits larger orbital interaction energies within the 2-thiouracil base and overall, contributing to its higher stability. []
Q9: How do modifications at the 5-position of 2-thiouridine affect its antiviral activity?
A10: Modifications at the 5-position of 2-thiouridine significantly impact its antiviral activity. 2'-Deoxy-5-alkyl-2-thiouridine analogues exhibit potent activity against varicella-zoster virus (VZV), with the 5-propyl derivative (TN-51) showing particularly promising results. These findings suggest that modifying the alkyl chain length at the 5-position can be a viable strategy for developing selective antiviral agents. []
Q10: What is the impact of halogenation at the 5-position of 2-thiouracil arabinoside on its antiviral activity?
A11: Halogenation at the 5-position of 2-thiouracil arabinoside generally leads to increased antiviral activity against herpes simplex virus (HSV) and VZV, with the exception of some anti-HSV activity. The observed trend indicates that substituting the halogen atom with one of higher molecular weight generally enhances potency against these viruses. []
Q11: How does the sulfur atom in 2-thiouridine affect its stability under oxidative stress?
A12: While 2-thiouridine contributes to tRNA stability and function under normal conditions, it becomes susceptible to desulfurization under oxidative stress. Exposure to hydrogen peroxide predominantly converts 2-thiouridine to 4-pyrimidinone nucleoside, significantly reducing its binding affinity to its complementary strand and potentially leading to strand scission. This susceptibility to oxidative damage highlights the importance of maintaining cellular redox balance for proper tRNA function. []
Q12: Can you elaborate on the use of gamma-toxin endonuclease for monitoring mcm5s2U modification in eukaryotic tRNAs?
A13: Gamma-toxin endonuclease from Kluvyeromyces lactis has emerged as a valuable tool for studying mcm5s2U modification in eukaryotic tRNAs. This enzyme specifically cleaves tRNAs containing mcm5s2U at the wobble position. By coupling this cleavage reaction with techniques like Northern blotting or quantitative PCR, researchers can monitor mcm5s2U levels in different tRNA species. This method has been successfully employed to investigate the evolutionary conservation of this modification and to validate the function of newly discovered enzymes involved in mcm5s2U biosynthesis. []
Q13: Are there any potential biomarkers associated with 2-thiouridine modifications in tRNA?
A14: While not directly linked to 2-thiouridine, the levels of s2U-modified tRNA, specifically s2U, have been proposed as a potential marker for sulfur availability in Bacillus subtilis. This suggests that the abundance of specific tRNA modifications might reflect cellular metabolic states and nutrient availability. []
Q14: What analytical techniques are commonly used to study 2-thiouridine and its derivatives?
A14: Various analytical techniques are employed to characterize and quantify 2-thiouridine and its derivatives. These include:
- UV Spectroscopy: Used to analyze the unique absorbance properties of 2-thiouridine compared to uridine. [, , ]
- NMR Spectroscopy: Provides detailed structural information, including sugar conformation, base pairing, and dynamics of oligonucleotides containing 2-thiouridine. [, , , , , , ]
- Mass Spectrometry: Enables the identification and quantification of 2-thiouridine and related modified nucleosides in tRNA. [, , ]
- High-Performance Liquid Chromatography (HPLC): Used to separate and quantify different modified nucleosides, including 2-thiouridine derivatives, from complex mixtures. [, ]
Q15: What are some valuable resources for researchers studying 2-thiouridine and tRNA modifications?
A15: Several resources facilitate research on 2-thiouridine and tRNA modifications, including:
- Software: Molecular modeling software packages, such as AMBER, allow researchers to simulate and analyze the structural and dynamic properties of RNA molecules containing 2-thiouridine using refined force field parameters. []
Q16: What are some of the key historical milestones in the study of 2-thiouridine?
A16: The study of 2-thiouridine has a rich history, with significant milestones including:
- Biosynthesis Elucidation: Unraveling the complex biosynthetic pathways involved in 2-thiouridine formation, identifying key enzymes and sulfur transfer mechanisms. [, , , , , , , ]
- Functional Characterization: Defining the crucial role of 2-thiouridine in codon recognition, translational fidelity, and overall tRNA stability. [, , , , , , ]
Q17: How has the study of 2-thiouridine fostered interdisciplinary research?
A17: The investigation of 2-thiouridine has stimulated collaborations across various scientific disciplines:
- Structural Biology: Utilizing X-ray crystallography and NMR spectroscopy to elucidate the structural basis for 2-thiouridine’s influence on RNA conformation and interactions. [, , ]
- Computational Chemistry: Developing and applying computational models and simulations to investigate the structural, dynamic, and energetic properties of 2-thiouridine-containing RNAs. [, ]
- Medicinal Chemistry: Exploring the potential of 2-thiouridine and its derivatives as antiviral agents and investigating their structure-activity relationships. [, ]
Avertissement et informations sur les produits de recherche in vitro
Veuillez noter que tous les articles et informations sur les produits présentés sur BenchChem sont destinés uniquement à des fins informatives. Les produits disponibles à l'achat sur BenchChem sont spécifiquement conçus pour des études in vitro, qui sont réalisées en dehors des organismes vivants. Les études in vitro, dérivées du terme latin "in verre", impliquent des expériences réalisées dans des environnements de laboratoire contrôlés à l'aide de cellules ou de tissus. Il est important de noter que ces produits ne sont pas classés comme médicaments et n'ont pas reçu l'approbation de la FDA pour la prévention, le traitement ou la guérison de toute condition médicale, affection ou maladie. Nous devons souligner que toute forme d'introduction corporelle de ces produits chez les humains ou les animaux est strictement interdite par la loi. Il est essentiel de respecter ces directives pour assurer la conformité aux normes légales et éthiques en matière de recherche et d'expérimentation.