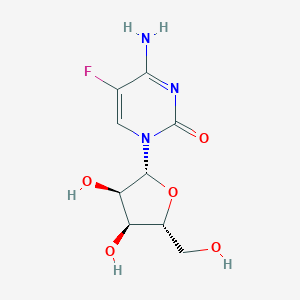
5-Fluorocytidine
Vue d'ensemble
Description
5-Fluorocytidine is a member of cytidines and has antiviral activity . It is a synthetic antimycotic compound that inhibits the maturation of the 45S ribosomal RNA precursor . It is also used as a prodrug that is converted to cytosine in vivo, and then incorporated into viral DNA by polymerase chain reactions .
Synthesis Analysis
5-Fluorocytidine is released through enzymatic cleavage of the carbamate and inhibits the growth of tumor cells . The batch synthesis of capecitabine generally requires long reaction times and significant quantities of Lewis acid . Bacterial amidohydrolases YqfB and D8_RL can activate novel prodrugs, and a significant decrease in the viability of HCT116 human colon cancer cell lines expressing either the YqfB or the D8_RL was observed after exposure to the novel prodrugs .
Molecular Structure Analysis
The molecular formula of 5-Fluorocytidine is C9H12FN3O5 . Its IUPAC name is 4-amino-1-((2R,5R)-3,4-dihydroxy-5-(hydroxymethyl)tetrahydrofuran-2-yl)-5-fluoropyrimidin-2(1H)-one .
Chemical Reactions Analysis
Bacterial amidohydrolases convert the non-toxic prodrugs into the therapeutic agent 5-fluorouridine in the HCT116 cell line when acting together with cellular cytidine deaminase . The products of 5-FU catabolism include α-fluoro-β-alanine (FBAL) and fluoroacetate .
Physical And Chemical Properties Analysis
5-Fluorocytidine has a molecular weight of 261.21 . It has a boiling point of 511.4±60.0 C at 760 mmHg and a melting point of 196-200C .
Applications De Recherche Scientifique
Gene-Directed Enzyme Prodrug Therapy
5-Fluorocytidine is used in gene-directed enzyme prodrug therapy, a strategy for cancer treatment. This therapy involves the delivery of a gene that encodes an enzyme capable of converting a prodrug into a potent cytotoxin exclusively in target cancer cells . Bacterial amidohydrolases YqfB and D8_RL have been found to activate novel prodrugs, leading to a significant decrease in the viability of HCT116 human colon cancer cell lines .
Enzyme-Prodrug System
5-Fluorocytidine, in combination with bacterial amidohydrolases, can serve as a promising enzyme-prodrug system for gene-directed enzyme prodrug therapy . This system can convert non-toxic prodrugs into the therapeutic agent 5-fluorouracil in target cells .
X-ray-Triggered Drug Delivery
5-Fluorocytidine has been functionalized with Au-Fe3O4 nanoheterodimers for X-ray-triggered drug delivery in breast tumor spheroids . This approach combines radiotherapy and chemotherapy, enhancing the therapeutic impact on multicellular tumor spheroids .
Synthesis of Anticancer Drugs
5-Fluorocytidine is used in the synthesis of new amide conjugates of hydroxycinnamic acids, which have shown slightly higher efficacy against primary BxPC-3 cells, a type of pancreatic cancer cell line .
Glycolytic Reprogramming
5-Fluorocytidine can affect the glycolytic metabolism due to oxygen deprivation inside multicellular tumor spheroids . The X-ray triggered release of 5-Fluorocytidine inhibits lactate secretion and thereby disturbs glycolytic reprogramming .
Cytotoxic Effects
5-Fluorocytidine exerts its cytotoxic effects on both healthy and tumor cells after their release into the cytosol . This makes it a potent tool in cancer research and treatment .
Mécanisme D'action
Target of Action
5-Fluorocytidine (5-FC), also known as Flucytosine, primarily targets susceptible fungal cells . It is most active against yeasts, including Candida, Torulopsis and Cryptococcus spp., and against the dematiaceous fungi causing chromomycosis (Phialophora and Cladosporium spp.) and Aspergillus spp .
Mode of Action
5-FC itself has no intrinsic antifungal activity. Its antimycotic activity results from the rapid conversion of 5-FC into 5-fluorouracil (5-FU) within susceptible fungal cells . This conversion is facilitated by the enzyme cytosine permease . Two major mechanisms of action have been elucidated: 5-FC is intrafungally converted into the cytostatic fluorouracil which undergoes further steps of activation and finally interacts as 5-fluorouridinetriphosphate with RNA biosynthesis thus disturbing the building of certain essential proteins .
Biochemical Pathways
The biochemical pathways affected by 5-FC involve both DNA and RNA synthesis. After being transported into susceptible fungi by cytosine permease, 5-FC is deaminated to 5-fluorouracil (5-FU) by cytosine deaminase . Following incorporation into DNA, 5-FU is converted to 5-fluorodeoxyuridylic acid monophosphate, which interferes with DNA synthesis . The other 5-FU activation pathway involves thymidine phosphorylase (dThdPase), which catalyzes the conversion of 5-FU to fluorodeoxyuridine (FUDR), and FUDR is then phosphorylated by thymidine kinase (TK) to FdUMP .
Pharmacokinetics
5-FC is well absorbed (75 to 90%) from the gastrointestinal tract . Following an oral dose of 2 grams, peak serum levels are reached after approximately 6 hours . The time to peak level decreases with continued therapy . It penetrates into body tissues well and is excreted mainly by the kidneys . In renal failure, major dose adjustments have to be made .
Result of Action
The result of 5-FC’s action is the inhibition of both DNA and RNA synthesis, leading to unbalanced growth and death of the fungal organism . This is achieved through the conversion of 5-FC into 5-FU within the fungal cells, which is then extensively incorporated into fungal RNA .
Action Environment
The action of 5-FC can be influenced by environmental factors such as the presence of other drugs. For instance, the most important drug interaction of 5-FC occurs with concomitant administration of 5-FC and nephrotoxic drugs, especially amphotericin B . This is because glomerular filtration plays a crucial role in 5-FC elimination, and drugs that impair this mechanism will decrease the elimination of 5-FC and thus prolong its half-life .
Safety and Hazards
Orientations Futures
Propriétés
IUPAC Name |
4-amino-1-[(2R,3R,4S,5R)-3,4-dihydroxy-5-(hydroxymethyl)oxolan-2-yl]-5-fluoropyrimidin-2-one | |
---|---|---|
Source | PubChem | |
URL | https://pubchem.ncbi.nlm.nih.gov | |
Description | Data deposited in or computed by PubChem | |
InChI |
InChI=1S/C9H12FN3O5/c10-3-1-13(9(17)12-7(3)11)8-6(16)5(15)4(2-14)18-8/h1,4-6,8,14-16H,2H2,(H2,11,12,17)/t4-,5-,6-,8-/m1/s1 | |
Source | PubChem | |
URL | https://pubchem.ncbi.nlm.nih.gov | |
Description | Data deposited in or computed by PubChem | |
InChI Key |
STRZQWQNZQMHQR-UAKXSSHOSA-N | |
Source | PubChem | |
URL | https://pubchem.ncbi.nlm.nih.gov | |
Description | Data deposited in or computed by PubChem | |
Canonical SMILES |
C1=C(C(=NC(=O)N1C2C(C(C(O2)CO)O)O)N)F | |
Source | PubChem | |
URL | https://pubchem.ncbi.nlm.nih.gov | |
Description | Data deposited in or computed by PubChem | |
Isomeric SMILES |
C1=C(C(=NC(=O)N1[C@H]2[C@@H]([C@@H]([C@H](O2)CO)O)O)N)F | |
Source | PubChem | |
URL | https://pubchem.ncbi.nlm.nih.gov | |
Description | Data deposited in or computed by PubChem | |
Molecular Formula |
C9H12FN3O5 | |
Source | PubChem | |
URL | https://pubchem.ncbi.nlm.nih.gov | |
Description | Data deposited in or computed by PubChem | |
DSSTOX Substance ID |
DTXSID60177964 | |
Record name | 5-Fluorocytidine | |
Source | EPA DSSTox | |
URL | https://comptox.epa.gov/dashboard/DTXSID60177964 | |
Description | DSSTox provides a high quality public chemistry resource for supporting improved predictive toxicology. | |
Molecular Weight |
261.21 g/mol | |
Source | PubChem | |
URL | https://pubchem.ncbi.nlm.nih.gov | |
Description | Data deposited in or computed by PubChem | |
CAS RN |
2341-22-2 | |
Record name | 5-Fluorocytidine | |
Source | CAS Common Chemistry | |
URL | https://commonchemistry.cas.org/detail?cas_rn=2341-22-2 | |
Description | CAS Common Chemistry is an open community resource for accessing chemical information. Nearly 500,000 chemical substances from CAS REGISTRY cover areas of community interest, including common and frequently regulated chemicals, and those relevant to high school and undergraduate chemistry classes. This chemical information, curated by our expert scientists, is provided in alignment with our mission as a division of the American Chemical Society. | |
Explanation | The data from CAS Common Chemistry is provided under a CC-BY-NC 4.0 license, unless otherwise stated. | |
Record name | 5-Fluorocytidine | |
Source | ChemIDplus | |
URL | https://pubchem.ncbi.nlm.nih.gov/substance/?source=chemidplus&sourceid=0002341222 | |
Description | ChemIDplus is a free, web search system that provides access to the structure and nomenclature authority files used for the identification of chemical substances cited in National Library of Medicine (NLM) databases, including the TOXNET system. | |
Record name | 5-Fluorocytidine | |
Source | EPA DSSTox | |
URL | https://comptox.epa.gov/dashboard/DTXSID60177964 | |
Description | DSSTox provides a high quality public chemistry resource for supporting improved predictive toxicology. | |
Record name | 5-Fluorocytidine | |
Source | European Chemicals Agency (ECHA) | |
URL | https://echa.europa.eu/information-on-chemicals | |
Description | The European Chemicals Agency (ECHA) is an agency of the European Union which is the driving force among regulatory authorities in implementing the EU's groundbreaking chemicals legislation for the benefit of human health and the environment as well as for innovation and competitiveness. | |
Explanation | Use of the information, documents and data from the ECHA website is subject to the terms and conditions of this Legal Notice, and subject to other binding limitations provided for under applicable law, the information, documents and data made available on the ECHA website may be reproduced, distributed and/or used, totally or in part, for non-commercial purposes provided that ECHA is acknowledged as the source: "Source: European Chemicals Agency, http://echa.europa.eu/". Such acknowledgement must be included in each copy of the material. ECHA permits and encourages organisations and individuals to create links to the ECHA website under the following cumulative conditions: Links can only be made to webpages that provide a link to the Legal Notice page. | |
Record name | 5-FLUOROCYTIDINE | |
Source | FDA Global Substance Registration System (GSRS) | |
URL | https://gsrs.ncats.nih.gov/ginas/app/beta/substances/VOR4X0D7WR | |
Description | The FDA Global Substance Registration System (GSRS) enables the efficient and accurate exchange of information on what substances are in regulated products. Instead of relying on names, which vary across regulatory domains, countries, and regions, the GSRS knowledge base makes it possible for substances to be defined by standardized, scientific descriptions. | |
Explanation | Unless otherwise noted, the contents of the FDA website (www.fda.gov), both text and graphics, are not copyrighted. They are in the public domain and may be republished, reprinted and otherwise used freely by anyone without the need to obtain permission from FDA. Credit to the U.S. Food and Drug Administration as the source is appreciated but not required. | |
Retrosynthesis Analysis
AI-Powered Synthesis Planning: Our tool employs the Template_relevance Pistachio, Template_relevance Bkms_metabolic, Template_relevance Pistachio_ringbreaker, Template_relevance Reaxys, Template_relevance Reaxys_biocatalysis model, leveraging a vast database of chemical reactions to predict feasible synthetic routes.
One-Step Synthesis Focus: Specifically designed for one-step synthesis, it provides concise and direct routes for your target compounds, streamlining the synthesis process.
Accurate Predictions: Utilizing the extensive PISTACHIO, BKMS_METABOLIC, PISTACHIO_RINGBREAKER, REAXYS, REAXYS_BIOCATALYSIS database, our tool offers high-accuracy predictions, reflecting the latest in chemical research and data.
Strategy Settings
Precursor scoring | Relevance Heuristic |
---|---|
Min. plausibility | 0.01 |
Model | Template_relevance |
Template Set | Pistachio/Bkms_metabolic/Pistachio_ringbreaker/Reaxys/Reaxys_biocatalysis |
Top-N result to add to graph | 6 |
Feasible Synthetic Routes
Q & A
Q1: How does 5-Fluorocytidine exert its antitumor effect?
A1: 5-Fluorocytidine acts as a prodrug, undergoing a series of enzymatic conversions to ultimately yield 5-Fluorouracil (5-FU) []. This active metabolite disrupts RNA synthesis and function, ultimately leading to cell death. [, ]
Q2: What makes the conversion of Capecitabine to 5-FU unique in tumor therapy?
A2: Capecitabine, a prodrug of 5-FU, relies on a three-enzyme cascade for activation, with the final step, conversion of 5′-deoxy-5-fluorouridine to 5-FU, primarily facilitated by thymidine phosphorylase (dThdPase) []. Tumor tissues often exhibit higher dThdPase levels compared to normal tissues, leading to localized 5-FU release and enhanced tumor selectivity [].
Q3: How does 5-Fluorocytidine impact ribosomal RNA maturation?
A3: Similar to other RNA-incorporated base analogs, 5-Fluorocytidine disrupts the maturation process of the 45S ribosomal RNA precursor, altering its electrophoretic mobility under non-denaturing conditions [].
Q4: Is the mechanism of action of 5-Fluorocytidine against viruses the same as its antitumor mechanism?
A4: While both activities stem from 5-Fluorocytidine's conversion to 5-FU, the precise mechanisms differ. Against viruses like HIV, the 5′-triphosphate metabolite of 5-Fluorocytidine acts as a competitive inhibitor of viral reverse transcriptase, halting viral DNA synthesis [].
Q5: What is the molecular formula and weight of 5-Fluorocytidine?
A5: The molecular formula of 5-Fluorocytidine is C9H12FN3O5, and its molecular weight is 261.20 g/mol. [, ]
Q6: How can 5-Fluorocytidine be characterized spectroscopically?
A6: Techniques such as 1H-NMR, 13C-NMR, FAB-MS, and elemental analysis have been employed to confirm the structure of 5-Fluorocytidine and its derivatives [, , ].
Q7: Are there specific challenges associated with formulating 5-Fluorocytidine?
A7: Research suggests that achieving optimal solubility and stability of 5-Fluorocytidine for pharmaceutical applications can be challenging. This has led to the exploration of prodrugs and alternative formulations to enhance its bioavailability and therapeutic efficacy [, , ].
Q8: How do structural modifications of 5-Fluorocytidine impact its anti-HIV activity?
A8: Research indicates that the presence of a 2′,3′-unsaturated sugar moiety, particularly with 3′-fluoro substitution, enhances the stability of the glycosyl bond in 5-Fluorocytidine analogs, leading to increased anti-HIV activity [].
Q9: Does changing the stereochemistry of 5-Fluorocytidine impact its activity?
A9: Yes, studies show that β-L-5-Fluorocytidine derivatives exhibit significantly enhanced antiviral activity against HBV compared to their β-D counterparts [].
Q10: Are there effective formulation strategies to improve the stability or bioavailability of 5-Fluorocytidine?
A10: Yes, synthesizing 5′-O-glucuronides of 5-Fluorocytidine has been explored as a prodrug strategy to enhance its stability and potentially reduce toxicity [].
Q11: How is Capecitabine metabolized in the human body?
A11: Capecitabine undergoes a three-step enzymatic conversion [, , ]. First, carboxylesterases, mainly in the liver, convert it to 5′-deoxy-5-fluorocytidine [, ]. Subsequently, cytidine deaminase, found in the liver and other tissues, transforms it into 5′-deoxy-5-fluorouridine [, , ]. Finally, thymidine phosphorylase, often in higher concentrations within tumors, activates it to 5-FU [, , ].
Q12: Does renal function impact the metabolism of capecitabine?
A12: Yes, a study observed a correlation between creatinine clearance (CLcr) and the metabolism of 5’-deoxy-5-fluorocytidine, a capecitabine metabolite. Lower CLcr was associated with increased conversion of 5’-deoxy-5-fluorocytidine to 5’-deoxy-5-fluorouridine, though the precise mechanism remains unclear [].
Q13: What is the oral bioavailability of β-d-2′,3′-Didehydro-2′,3′-Dideoxy-5-Fluorocytidine (D-D4FC)?
A13: Studies in rhesus monkeys determined the oral bioavailability of D-D4FC to be approximately 41% [, ].
Q14: Have there been any in vivo studies on the antitumor efficacy of 5′-deoxy-5-fluorocytidine conjugates?
A14: Yes, in vivo studies using a human bladder cancer cell line (T24) demonstrated that forced expression of cytidine deaminase increased sensitivity to 5′-deoxy-5-fluorocytidine and capecitabine [].
Q15: How does the antitumor activity of N4-Trimethoxybenzoyl-5′-deoxy-5-fluorocytidine (Ro 09-1390) compare to its parent drug, 5′-deoxy-5-fluorouridine?
A15: Studies using transplantable tumor models found that Ro 09-1390 exhibited antitumor efficacy comparable to 5′-deoxy-5-fluorouridine, and both were significantly more effective than 5-fluorouracil and tegafur [].
Q16: Does resistance develop to β-l-2′,3′-dideoxy-2′,3′-didehydro-5-fluorocytidine (β-l-Fd4C) in treating chronic hepatitis B?
A16: While β-l-Fd4C demonstrates potent antiviral effects against woodchuck hepatitis virus (WHV), the persistence of covalently closed circular DNA contributes to the lack of complete eradication of infected hepatocytes and the potential for viral relapse after treatment cessation [].
Q17: Can HIV-1 develop resistance to L-nucleoside analogs?
A17: Yes, in vitro studies have shown that HIV-1 can develop resistance to L-nucleoside analogs, including β-L-2′,3′-didehydro-2′,3′-dideoxy-5-fluorocytidine (β-L-D4FC), through mutations like V184 and K/R65 in the reverse transcriptase gene [].
Q18: What are the potential side effects of N4-Trimethoxybenzoyl-5′-deoxy-5-fluorocytidine (Ro 09-1390)?
A18: Although Ro 09-1390 demonstrated comparable antitumor activity to 5′-deoxy-5-fluorouridine, it exhibited significantly less toxicity to the intestinal tract and a less severe immunosuppressive effect, suggesting a potentially improved safety profile [].
Q19: Does β-l-2′,3′-dideoxy-5-fluorocytidine impact mitochondrial DNA synthesis?
A19: Studies indicate that β-l-2′,3′-dideoxy-5-fluorocytidine does not inhibit mitochondrial DNA synthesis at concentrations up to 10 μM, suggesting a lower risk of mitochondrial toxicity compared to some other nucleoside analogs [].
Q20: Are there cardiac concerns with capecitabine therapy?
A20: While generally well-tolerated, rare cases of cardiac toxicity, such as chest pain potentially linked to coronary spasm, have been reported in patients receiving capecitabine therapy [].
Q21: What analytical methods are employed to measure Capecitabine and its metabolites in biological samples?
A21: Several techniques have been developed for quantifying Capecitabine and its metabolites in plasma, including: * High-performance liquid chromatography coupled with tandem mass spectrometry (HPLC-MS/MS), offering high sensitivity and selectivity for simultaneous determination of multiple analytes. [, , ] * Ultra-high performance liquid chromatography coupled with tandem mass spectrometry (UPLC-MS/MS), enabling faster analysis times while maintaining sensitivity and selectivity. []
Q22: Are there analytical methods available to determine the levels of β-l-2′,3′-Dideoxy-5-Fluorocytidine in biological samples?
A22: Yes, β-l-2′,3′-Dideoxy-5-Fluorocytidine levels in plasma and urine samples can be determined using high-pressure liquid chromatography (HPLC) []. This technique allows for the separation and quantification of the drug, providing valuable data for pharmacokinetic analysis.
Avertissement et informations sur les produits de recherche in vitro
Veuillez noter que tous les articles et informations sur les produits présentés sur BenchChem sont destinés uniquement à des fins informatives. Les produits disponibles à l'achat sur BenchChem sont spécifiquement conçus pour des études in vitro, qui sont réalisées en dehors des organismes vivants. Les études in vitro, dérivées du terme latin "in verre", impliquent des expériences réalisées dans des environnements de laboratoire contrôlés à l'aide de cellules ou de tissus. Il est important de noter que ces produits ne sont pas classés comme médicaments et n'ont pas reçu l'approbation de la FDA pour la prévention, le traitement ou la guérison de toute condition médicale, affection ou maladie. Nous devons souligner que toute forme d'introduction corporelle de ces produits chez les humains ou les animaux est strictement interdite par la loi. Il est essentiel de respecter ces directives pour assurer la conformité aux normes légales et éthiques en matière de recherche et d'expérimentation.