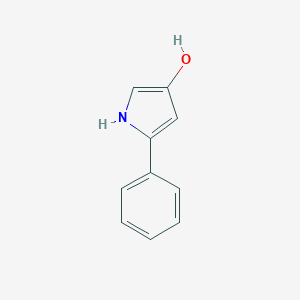
3-Hydroxy-5-phénylpyrrole
Vue d'ensemble
Description
3-Hydroxy-5-phenylpyrrole is a phenolic compound with the molecular formula C10H9NO and a molecular weight of 159.18 g/mol . It is characterized by a pyrrole ring substituted with a hydroxyl group at the third position and a phenyl group at the fifth position. This compound is found in the essential oils of various plants and has been studied for its potential biological activities .
Applications De Recherche Scientifique
3-Hydroxy-5-phenylpyrrole has a wide range of scientific research applications, including:
Chemistry: It is used as a reference standard in pharmaceutical testing and as a building block in organic synthesis.
Medicine: Research is ongoing to explore its potential therapeutic applications, including its role in drug development.
Industry: It is used in the production of dyes and other chemical products.
Mécanisme D'action
Target of Action
3-Hydroxy-5-phenylpyrrole (HOPPy) is primarily used for detecting the presence of hydrolytic analytes . Its primary targets are enzymes such as esterases, leukocytes, and proteases . These enzymes play crucial roles in various biological processes, including metabolism and immune response.
Mode of Action
It is known that hoppy interacts with its targets (hydrolytic enzymes) and causes changes that allow for the detection of these enzymes . This interaction is likely due to the structural characteristics of HOPPy, which enable it to bind to the active sites of these enzymes.
Result of Action
The primary result of HOPPy’s action is the detection of hydrolytic analytes. It is used in research settings to detect the presence of enzymes like esterases, leukocytes, and proteases . The detection of these enzymes is especially important in medical diagnostics .
Analyse Biochimique
Biochemical Properties
3-Hydroxy-5-phenylpyrrole is used for detecting the presence of hydrolytic analytes, such as esterases, leukocytes, and proteases . The detection of leukocytes is especially important in medical diagnostics .
Cellular Effects
It has been shown to reduce appetite and increase oxygen consumption in animal models , suggesting that it may influence cellular metabolism and energy utilization.
Molecular Mechanism
Its ability to detect hydrolytic analytes suggests that it may interact with enzymes such as esterases, leukocytes, and proteases .
Metabolic Pathways
It is known to be a phenolic compound found in the essential oils of many plants , suggesting that it may be involved in the metabolism of these oils.
Méthodes De Préparation
Synthetic Routes and Reaction Conditions
3-Hydroxy-5-phenylpyrrole can be synthesized through several methods. One common approach involves the reaction of fatty acids with isovaleric acid in the presence of a catalyst . Another method includes the supercritical extraction technique from plant materials . Additionally, it can be extracted from bitter herbs such as gentian or wormwood .
Industrial Production Methods
Industrial production of 3-Hydroxy-5-phenylpyrrole typically involves large-scale extraction from natural sources or chemical synthesis using optimized reaction conditions to ensure high yield and purity. The specific industrial methods may vary depending on the desired application and available resources.
Analyse Des Réactions Chimiques
Types of Reactions
3-Hydroxy-5-phenylpyrrole undergoes various chemical reactions, including:
Oxidation: The hydroxyl group can be oxidized to form corresponding ketones or aldehydes.
Reduction: The compound can be reduced to form different derivatives with altered functional groups.
Substitution: The phenyl group can undergo electrophilic aromatic substitution reactions.
Common Reagents and Conditions
Oxidation: Common oxidizing agents include potassium permanganate and chromium trioxide.
Reduction: Reducing agents such as sodium borohydride or lithium aluminum hydride are typically used.
Substitution: Electrophilic aromatic substitution reactions often involve reagents like halogens or nitrating agents under acidic conditions.
Major Products Formed
The major products formed from these reactions depend on the specific reagents and conditions used. For example, oxidation may yield ketones or aldehydes, while reduction can produce alcohols or amines. Substitution reactions can introduce various functional groups onto the phenyl ring.
Comparaison Avec Des Composés Similaires
Similar Compounds
5-Phenyl-1H-pyrrol-3-ol: Similar in structure but lacks the hydroxyl group at the third position.
3-Hydroxy-5-methylpyrrole: Similar in structure but has a methyl group instead of a phenyl group at the fifth position.
Uniqueness
3-Hydroxy-5-phenylpyrrole is unique due to its specific substitution pattern, which imparts distinct chemical and biological properties. Its combination of a hydroxyl group and a phenyl group on the pyrrole ring makes it a valuable compound for various research and industrial applications.
Propriétés
IUPAC Name |
5-phenyl-1H-pyrrol-3-ol | |
---|---|---|
Source | PubChem | |
URL | https://pubchem.ncbi.nlm.nih.gov | |
Description | Data deposited in or computed by PubChem | |
InChI |
InChI=1S/C10H9NO/c12-9-6-10(11-7-9)8-4-2-1-3-5-8/h1-7,11-12H | |
Source | PubChem | |
URL | https://pubchem.ncbi.nlm.nih.gov | |
Description | Data deposited in or computed by PubChem | |
InChI Key |
BMJFHUYFKGXBCA-UHFFFAOYSA-N | |
Source | PubChem | |
URL | https://pubchem.ncbi.nlm.nih.gov | |
Description | Data deposited in or computed by PubChem | |
Canonical SMILES |
C1=CC=C(C=C1)C2=CC(=CN2)O | |
Source | PubChem | |
URL | https://pubchem.ncbi.nlm.nih.gov | |
Description | Data deposited in or computed by PubChem | |
Molecular Formula |
C10H9NO | |
Source | PubChem | |
URL | https://pubchem.ncbi.nlm.nih.gov | |
Description | Data deposited in or computed by PubChem | |
DSSTOX Substance ID |
DTXSID70403206 | |
Record name | 3-HYDROXY-5-PHENYLPYRROLE | |
Source | EPA DSSTox | |
URL | https://comptox.epa.gov/dashboard/DTXSID70403206 | |
Description | DSSTox provides a high quality public chemistry resource for supporting improved predictive toxicology. | |
Molecular Weight |
159.18 g/mol | |
Source | PubChem | |
URL | https://pubchem.ncbi.nlm.nih.gov | |
Description | Data deposited in or computed by PubChem | |
CAS No. |
100750-40-1 | |
Record name | 3-HYDROXY-5-PHENYLPYRROLE | |
Source | EPA DSSTox | |
URL | https://comptox.epa.gov/dashboard/DTXSID70403206 | |
Description | DSSTox provides a high quality public chemistry resource for supporting improved predictive toxicology. | |
Synthesis routes and methods
Procedure details
Retrosynthesis Analysis
AI-Powered Synthesis Planning: Our tool employs the Template_relevance Pistachio, Template_relevance Bkms_metabolic, Template_relevance Pistachio_ringbreaker, Template_relevance Reaxys, Template_relevance Reaxys_biocatalysis model, leveraging a vast database of chemical reactions to predict feasible synthetic routes.
One-Step Synthesis Focus: Specifically designed for one-step synthesis, it provides concise and direct routes for your target compounds, streamlining the synthesis process.
Accurate Predictions: Utilizing the extensive PISTACHIO, BKMS_METABOLIC, PISTACHIO_RINGBREAKER, REAXYS, REAXYS_BIOCATALYSIS database, our tool offers high-accuracy predictions, reflecting the latest in chemical research and data.
Strategy Settings
Precursor scoring | Relevance Heuristic |
---|---|
Min. plausibility | 0.01 |
Model | Template_relevance |
Template Set | Pistachio/Bkms_metabolic/Pistachio_ringbreaker/Reaxys/Reaxys_biocatalysis |
Top-N result to add to graph | 6 |
Feasible Synthetic Routes
Q1: What is the significance of the tosylalanine ester of 3-hydroxy-5-phenylpyrrole (Tos-Ala-OPPy)?
A: Tos-Ala-OPPy serves as a substrate for human leukocyte elastase (HLE) and is utilized in diagnostic reagent strips (e.g., Ames LEUKOSTIX) to detect HLE in urine, indicating potential urinary tract infections []. Kinetic studies revealed that Tos-Ala-OPPy exhibits high reactivity with HLE, with deacylation being the rate-limiting step in its hydrolysis [].
Q2: How does 3-hydroxy-5-phenylpyrrole (HOPPy) participate in the colorimetric detection of HLE?
A: After HLE cleaves Tos-Ala-OPPy, the released HOPPy reacts with a diazonium salt, 4-diazo-3-hydroxy-1-napthylsulfonate (HONapN+2), present in the reagent strip matrix. This coupling reaction generates a purple color, signaling the presence of HLE []. The rate of this colorimetric reaction is influenced by the substituents on the naphthalene ring of the diazonium salt and the buffer system used [].
Q3: What interesting oxidation product forms from diethyl 1-methyl-3-hydroxy-5-phenylpyrrole-2,4-dicarboxylate in basic conditions?
A: In the presence of aqueous base and oxygen, diethyl 1-methyl-3-hydroxy-5-phenylpyrrole-2,4-dicarboxylate undergoes oxidation, yielding two products: ethyl 1-methyl-2-oxo-3-hydroxy-5-phenyl-3-pyrroline-4-carboxylate (9) and ethyl 1-methyl-2,3-dioxo-5-phenyl-4-pyrroline-4-carboxylate (10). These products likely arise from the decomposition of a common intermediate, diethyl 1-methyl-2-hydroperoxy-3-oxo-5-phenyl-4-pyrroline-2,4-dicarboxylate [].
Avertissement et informations sur les produits de recherche in vitro
Veuillez noter que tous les articles et informations sur les produits présentés sur BenchChem sont destinés uniquement à des fins informatives. Les produits disponibles à l'achat sur BenchChem sont spécifiquement conçus pour des études in vitro, qui sont réalisées en dehors des organismes vivants. Les études in vitro, dérivées du terme latin "in verre", impliquent des expériences réalisées dans des environnements de laboratoire contrôlés à l'aide de cellules ou de tissus. Il est important de noter que ces produits ne sont pas classés comme médicaments et n'ont pas reçu l'approbation de la FDA pour la prévention, le traitement ou la guérison de toute condition médicale, affection ou maladie. Nous devons souligner que toute forme d'introduction corporelle de ces produits chez les humains ou les animaux est strictement interdite par la loi. Il est essentiel de respecter ces directives pour assurer la conformité aux normes légales et éthiques en matière de recherche et d'expérimentation.